“When you cut your teeth on other worlds,” Carl Sagan once noted, “you gain a perspective about the fragility of planetary environments and about what other, quite different, environments are possible.”71 Sagan points to James Hansen’s research of Venus that led to early climate models to predict how greenhouse gases had been trapped in its atmosphere and might similarly impact Earth’s climate, as well as Mario Molina and Sherwood Rowland’s investigation of chlorine and fluorine molecules in Venus’s atmosphere that led to an understanding of the threat of CFCs to Earth’s ozone layer. These investigations of Venus revealed that Earth’s dynamic biosphere could lose its ability to support life.
Lovelock has expressed his concern not so much that our carbon footprint will destroy the Earth, but that warming of Earth’s atmosphere could mean the end of our civilization as we know it. Suggesting we make plans for “sustainable retreat,” Lovelock at the age of eighty-nine comments, “It’s really a question of. . . where we will get our food, water. How we will generate energy.”72 This is not a new position for Lovelock. Even from the beginning stages of Gaia Theory, Steven Dick and James Strick comment, “He realized that the gases that living organisms most actively affect, especially carbon dioxide, methane, oxygen and water vapor, are just those gases that most dramatically shape the climate of a planet.”73 “I can only guess the details of the warm spell due,” wrote Lovelock in 1988. “Will Boston, London, Venice, and the Netherlands vanish beneath the sea? Will the Sahara extend to cross the equator?”74 He’s not being facetious.
In The Ice Chronicles, scientist Paul Mayewski and science writer Frank White discuss the findings from the Greenland Ice Sheet Project 2’s (GISP2) ice cores drilled in central Greenland in 1990. The cores capture a 100,000-year record of the chemical composition of Earth’s atmosphere and a picture of the rate of thinning of Greenland’s glaciers. Of the book, Lynn Margulis noted, “The story of how international science obtained this fund of Pleistocene data from the central Greenland ice sheet reads like a novel.”75 However, this text isn’t some action-adventure tale of humans against nature, but a serious assessment of changes in atmospheric composition over long time scales. Captured deep in the Greenland ice are tiny air bubbles that serve as time capsules from thousands of years ago. Scientists can analyze the air in the bubbles to determine the composition of Earth’s atmosphere in ages past. Mayewski and White assert unequivocally that increases in methane and carbon dioxide produced through industry, agriculture, and mass transport are dramatically warming our planet’s atmosphere. They write: “As with CO2, methane is increasing at a rate that has never before been seen, and, as with CO2, the increase correlates strongly with rising temperatures. . . . The result is that we are having a profound impact on the climate system.” Mayewski and White note that the ice core data indicate that ecosystems on a global scale can be “easily and quickly disturbed.”76 Climatologists are concerned that global warming could cause the collapse of the world’s ice sheets, which in turn would produce an unprecedented rise in sea level. In 2011, British glaciologist Alun Hubbard reported that an expanse of glacial ice estimated at twice the size of Manhattan was on the brink of breaking free from Greenland. The previous year a stretch of ice four times the size of Manhattan, spanning 112 square miles, broke off the Greenland Ice Sheet and was adrift at sea in large chunks. “The freshwater stored in this ice island could keep the Delaware or Hudson rivers flowing for more than two years,” estimated Andreas Muenchow at the University of Delaware. “It could also keep all U. S. public tap water flowing for 120 days.”77 Though Muenchow emphasizes that ocean scientists, glaciologists, and climatologists cannot yet predict the effects of melting glacial ice, he is nevertheless concerned, and he’s not alone. The 2001 Amsterdam Declaration on Global Change reads:
Global change cannot be understood in terms of a simple cause – effect paradigm. Human-driven changes cause multiple effects that cascade through the Earth System in complex ways. These effects interact with each other and with local – and regional-scale changes in multidimensional patterns that are difficult to understand and even more difficult to predict. . . . In terms of some key environmental parameters, the Earth System has moved well outside the range of the natural variability exhibited over the last half million years at least. The nature of changes now occurring simultaneously in the Earth System, their magnitudes and rates of change are unprecedented. The Earth is currently operating in a no-analogue state.78
It’s no coincidence that Gaia Theory emerged in the late 1960s and early 1970s at the height of the Space Age, precisely as space travel and satellite observation began offering consistent and detailed global views of Earth (figure 2.5). NASA’s Landsat 1, launched in 1972, was the first satellite dedicated solely to Earth observation, particularly of landmasses.79 Nor is it surprising that
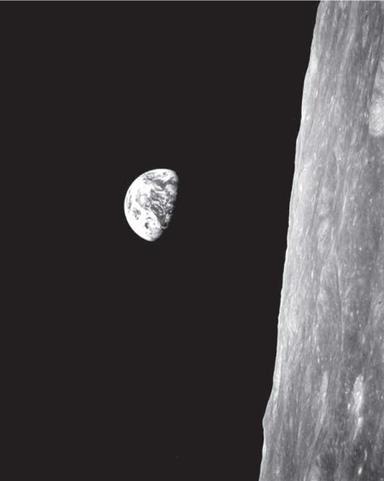
Figure 2.5. Earthrise in the orientation from which the Apollo 8 crew saw it from lunar orbit. In zero gravity, the normal senses of up, down, and the horizon have no particular meaning. Just two dozen astronauts have ever experienced the extraordinary sight of their home planet rising over the horizon of another world (NASA/Apollo 8).
|
the original cover of Lovelock’s book Gaia: A New Look at Life on Earth (1979) was illustrated with Apollo 17’s Whole Earth photograph, considered among photo historians as the most reproduced image in history. While “Gaia theory forces a planetary perspective,” as Lovelock points out, that invaluable perspective was only possible with a serious commitment to space exploration. “It took the view of the Earth from space,” writes Lovelock, “either directly through the eyes of an astronaut, or vicariously through visual media, to let us sense a planet on which living things, the air, the oceans, and rocks all combine in one as Gaia.”80
By 1983, NASA had established the Earth System Sciences Committee (ESSC) to publish recommendations for a concerted science program in global Earth studies and satellite observing programs. NASA’s planetary science and recent Earth observing missions have offered scientists their best understanding yet of the complex and interconnected Earth systems that sustain our biosphere. Several missions are specifically focused on understanding and mitigating, if possible, the effects of industrialized human activity.
NASA’s GOES satellites offer real-time data regarding Earth’s weather systems. The Aqua mission measures all aspects of Earth’s water cycle in the solid, liquid, and gas states as well as global water temperatures, vegetation cover, and phytoplankton, which construct their shells from carbon dioxide and deposit that carbon onto the seafloor when they expire. The joint NASA and Argentinian Aquarius mission catalogs the salinity of Earth’s oceans, while NASA’s Aura mission monitors changes to Earth’s ozone layer. In collaboration with the German Aerospace Center, NASA supports the GRACE mission, which has observed a large drawdown of fresh water globally, from Northern Africa to the large agricultural San Joaquin valley in California.81 NASA and the French Space Agency together support the CALIPSO mission to track airborne particles or aerosols to determine their impact on global climate. These are only a handful of the varied and complex missions NASA supports in observing Earth’s biological, hydrological, and geochemical systems. What we have learned from missions like these not only helps us to better understand climate change and its impact on life on Earth but also has prepared us to search for life on moons in the outer solar system and on exoplanets orbiting nearby stars. When telescopic detectors become sensitive enough, astrobiologists will scour the spectra of exoplanets light-years away searching for the biomarkers of oxygen or ozone, as these gases have no other significant source on Earth than its living organisms.
In 2006, on the thirtieth anniversary of the Viking Mission, Joel Levine, principal investigator of the proposed Mars Ares mission to fly a robotic plane over Mars to search for evidence of life, noted that scholars are still researching Viking’s nearly 60,000 images, which remain “one of the most exciting, one of the most productive data sets we have ever obtained.”82 Venus and Mars, the closest worlds to Earth, retain their allure and mystery. Venus is a near twin of the Earth, our true sister planet, yet a runaway greenhouse effect has heated it to the point where life isn’t possible. It’s a salutary reminder of what might happen if we don’t get our house in order in terms of carbon emissions. We wince and turn away. Mars, meanwhile, is the lightweight cousin of the Earth. In 2009, scientists at the India Space Research Organization (ISRO) reported having discovered thriving in Earth’s stratosphere three previously unidentified species of bacteria, despite consistent exposure to ultraviolet radiation that usually kills such organisms. Given that conditions in the Earth’s stratosphere closely resemble the atmosphere on Mars, the finding profoundly recalls Viking’s controversial experiments conducted on Martian soil decades ago. If there is biology on Mars, it leads a tenuous existence in hospitable pockets below the surface. Until we definitively discover life, either on Mars or elsewhere, we invest these worlds with our full imagination, and project onto them our hopes, fears, and longings, anticipating the time when our isolation ends and we find companionship in the cosmos—if only in a colony of microbes.