When Armstrong and Aldrin arrived at Tranquillity Base, their flight plan gave them a rest period before they were to embark on their historic moonwalk. NASA’s operational conservatism had not wanted to load them up with too much in one day. But many believed that the crew would be too keyed up to rest at that point and there were tentative plans to bring their EVA forward. One hour after landing. Armstrong announced his decision. “Our recommendation at this point is planning an EVA, with your concurrence, starting about eight o’clock this evening. Houston time. That is about three hours from now. We will give you some lime to think about that."
Charlie Duke promptly gave Houston’s reply. “We thought about it; wre will support it. We’re Go at that time.’’
Aflcr the mission, Armstrong explained their thinking. “There were two factors that we thought might influence that decision. One was the spacecraft systems and any abnormalities that we might have that we’d want to work on; and the second was our adaptation to one-sixth g and whether we thought more time in one-sixth g before starting the EVA would be advantageous or disadvantageous at that point. Basically, my personal feeling was that the adaptation to one-sixth g was very rapid and [it] was very pleasant, easy to work in. and I thought at the time that we were ready to go right ahead into the surface work and [so I] recommended that."
For the most part, crews had their first surface EVA on the day of landing. Apollo 16 was one exception. The 6-hour delay in its landing had changed mission control’s view. “You probably gathered that we want y’all to sleep first," said Jim Irwin in Houston.
“That suits us," said Duke, not entirely honestly. “Jim. I feel exactly like I thought I was [going to feel], I really want to get out, hut I think that discretion is the better part of the valour here.’’
Decades later. Duke regretted the decision. “As a hindsight observation, it wasn’t a good idea. I think we should have gotten out first. I had a tough time getting to sleep. My mind wras just racing and I wanted to get out. I was thinking about all that was coming up; and the excitement had just passed; and. you know, my mind was just whirling. 1 think wn had so much adrenaline pumping we could have gone, probably two days – forty-eight hours – without any problem. So, looking back, I think we made a mistake. But it’s done."
The other exception to EVA on landing day was Apollo 15. They had a SEVA!
SEVA
David Scott’s flight was the first J-mission, the first to have a rover and the first where science defined the mission. Back in 1964, when geology training began for the prospective lunar explorers, Scott’s teachers spotted that, more than the other jet – jockeys that passed through their hands, he possessed a mind that was receptive to scientific enquiry. When his Moon mission came up. his enthusiasm and excitement for Apollo’s scientific quest easily transferred to the other members of his crew, and to those subsequent members of the J-mission crews who, like him, came from a test – pilot background. Don Wilhelms, who played a major part in transforming Apollo into a hunt for geological answers, described Scott, Jim Irwin and Л1 Worden as "the geologic crew”.
Scott had been impressed by the teachings of geologists like Caltech professor Lee Silver and USGS staffer Gordon Swann who had been brought in to give the crews training in field geology, as distinct from the previous classroom instruction. When approaching a new’ geological site, one of Silver’s techniques was to find a high spot, even the vehicle that had brought them there if needs be, to gain an overview of the site before digging into the detail of stones and bedrock. This site survey gave the bigger picture; what the major rock exposures were, what type of rocks they held and at w’hat angle the layers of rock dipped. Scott decided that since there were no plans for an EVA on the day of their landing, he w’ould make a stand-up extravehicular activity (SEVA) instead.
The arguments to convince managers that a SEVA was worthwhile included a need to maintain the crew’s circadian rhythm. At the time, it was felt that a full 7- hour EVA after landing would have meant an excessively long day. Yet. to sleep so soon after arrival would lead to a shorter than normal day. The SEVA would fill the gap and help to release the excitement they would naturally feel at having landed on the Moon. Also, Apollo 15 s northerly site had not been well mapped by the Lunar Orbiter probes whose high-resolution imagery had been expended near the equator. In particular, no one really knew w’hether Iladley provided a suitable surface for the rover, and if significant problems became visible from up top then his descriptions would be useful in revising the planned drives.
From Scott’s point of view, the best reason for the SEVA was the science, as he later recalled. "To be able to stand there and just look at all that stuff. I mean, that was just a mindblower to be able to just stand up there and gaze around and report what you saw% knowing full w’ell that Lee Silver and Gordon Sw’ann and the guys in the Backroom are listening to every word.”
As well as the SEVA, Scott and the geologists wanted to take a telephoto lens. "We spent a lot of time and energy justifying the 500-mm lens,” remembered Scott. "And the final trade-off was in abort propellant. They reduced the amount of abort propellant on the landing by witatever the mass of the telephoto lens w’as. There was a lot of scepticism on whether it would be useful at all. But, gosh, you go out in the field with a bunch of geologists and you can’t get to the mountain and it becomes obvious that a telephoto picture is a lot better than nothing.”
Once he had got his head out of the overhead hatch. Scott began to photograph the virgin site using a 60-mrn lens to take an all-round panorama and then his telephoto lens to capture features of special interest. With that out of the way, he began what, according to Wilhelms, ranked as "the best geological description by an astronaut on the Moon.”
"All of the features around here are very smooth,” reported Scott. "The tops of the mountains are rounded off. There are no sharp jagged peaks or no large boulders apparent anywhere.”
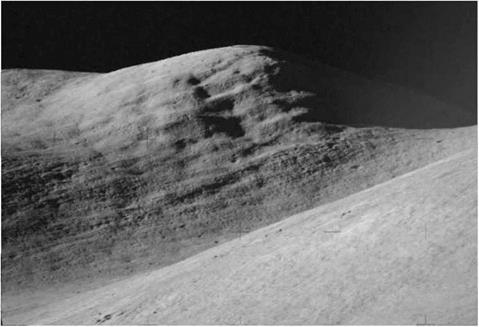
Silver Spur, as photographed by David Scott during his SEVA using a 500-mrn lens. (NASA) " " "
|
That was good news. They would have little difficulty driving the rover across the landscape as planned. Scott worked his way around the view, describing everything he saw while giving clock-face references with respect to the LM’s west-facing aspect. "As I look on down to my seven o’clock, I guess I see Index Crater here [in] the near field. But, back up on Hadley Delta, to the east [at Silver Spur], why, again I can see a smooth surface. However, I can see lineaments. I’ll take a picture for you.”
The Moon’s immense antiquity drapes almost all its bedrock with a thick blanket of beat-up, pulverised rock. Since bedrock is what geologists like the most, by virtue of it being in situ, any sign of it on the lunar surface gets their attention, especially when they see layering. Scott’s lineaments, on the feature they had happily named Silver Spur for their geological mentor, appeared to be such layering.
"There appear to be lineaments or lineations dipping to the northeast, parallel [to each other]. And they appear to be, maybe, three per cent to four per cent of the total elevation of the mountain, almost uniform [spacing]. I can’t tell whether it’s structure or internal stratigraphy or what. But there are definite linear features there, dipping to the northeast, at about, oh, I’d say 30 degrees.”
Silver Spur was too far away to visit, but with his telephoto lens Scott was able to gain clear images of the structures for the geologists.
For the two later missions, the justifications for a SEVA lessened and it was dropped. Scott would be the only commander to look out of the top of his LM in what Jim Irwin likened to Desert Fox in his Panzer.
SUITING UP
If a crew intended to leave the lunar module soon after landing, their preparations were reduced by virtue of being already suited. In fact, for the first three landings, suits were never removed while on the Moon. Their task primarily involved changing over from the LM’s oxygen and coolant water to that supplied from their back pack, the portable life support system (PLSS, pronounced ‘plies’). Once they had put their helmets and gloves back on, there were a large number of checks to be made.
The LM provided very little room for two crewmen wearing suits with PLSSs attached, so one of the first tasks was to lay out all the items they would need at positions where they could get to them without undue difficulty. They checked their zippers, the double locks that would hold their gloves and helmets in place, then positioned themselves to put their back packs on. The sequence of steps in the process was very carefully choreographed. Among all the unstowing of cameras, boots and other paraphernalia was one important task; to deploy a VHF antenna on the outside of the LM by turning a crank. One of the crew had to manoeuvre to the back of the cabin to get at it. When crews had eight hours or more hard work outside while sealed inside a suit, some food was welcome while an occasional drink was essential, so for the later missions, bags of water and a food bar would be installed within the neckring. Then coatings of anti-fog treatment would be applied to the inside of their helmets.
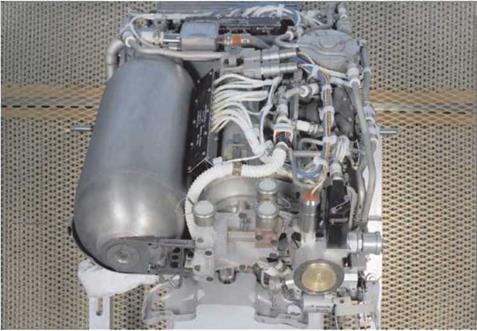
A PLSS with its covers removed. (NASA)
|
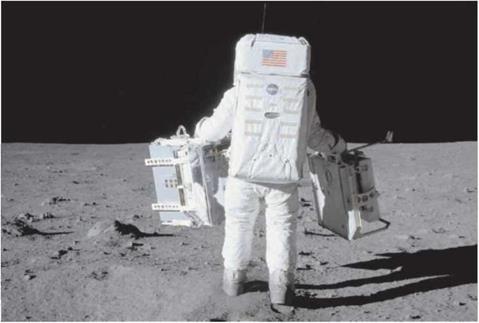
Buzz Aldrin carries science equipment across the lunar surface. On his back is his OPS (with the US flag) and PLSS (the larger unit below the OPS). (NASA)
|
Before they added their oxygen purge system (OPS) to the top of the back packs, a check was made of the pressure in the oxygen tanks within. Each OPS had two spherical tanks with the gas inside stored at an extremely high pressure. A small gauge was provided to check its value. Depending on how a crewman’s PLSS or suit had failed, the OPS would provide at least 30 minutes of emergency oxygen and cooling by the regulated discharge of the contents of its tanks. In fact, the OPS carried more than three times as much oxygen as did the PLSS itself but the latter was merely recycling the gas in a loop and topping it up as necessary as it was consumed.
As the Apollo 14 crew worked through their suiting up procedures, they reached the point where they had to don the PLSS. Mission control had them call out where in the checklist they were. “Okay, Houston,” called Ed Mitchell. “We’re at that point where we hand the real PLSS out and get the lightweight one.”
Once again, the crews could not help but compare their amazing experience on the surface of the Moon with the endless rehearsals they had put in before the flight. During their training exercises or when they had checked out Ant ares itself, they had used a real PLSS up to the point of donning it, but the back packs were uncomfortably heavy in Earth’s gravity and unwieldy in the tight space of the LM cabin. To avoid damaging flight hardware, the real PLSS would be exchanged for a lightweight mock-up. Ron Blevins was an instructor who would typically make the exchange.
“Roger,” laughed Capcom Bruce McCandless. “I’ll have Ron come on up the ladder.”
Crews did find the LM cabin to be a much more pleasant environment when the one-sixth-g of the Moon ruled the physics of their work. “The only time I found the lunar module really confining was when we started moving the PLSSs around,” remembered Charlie Duke. “We move one of the PLSSs on the floor between us, and we pick it up and put it somewhere else. We’d done that in training and John just struggled to get that thing up, because it was heavy! You know, 155 pounds [70 kg]. They had a lightweight mock-up which 1 think was a little bit lighter. But up there, in that one-sixth g, boy, he just picked it up with one hand. It was just tremendous. It made you feel like Superman.”
With the OPS attached to the top of the PLSS, connections were made between the two, including a feed to the antenna on top through which the crew would keep in touch with each other and Earth. Then they would don the PLSS. “Getting the PLSSs on was very difficult,” recalled Gene Cernan. “It was a two-man operation. The PLSSs were strapped to the side walls, at working height, and you had to back up against yours and the other guy would then unhook it from the wall and help you get it strapped to your back. The LM was so small that it was a very difficult operation, even in soft (unpressurised) suits.”
To control and monitor most of the functions of the suit, a remote control unit (RCU) was attached to the chest. It had a quantity gauge for oxygen; switches that controlled their water pump and fan assembly, and their communications; and there were flags that indicated the status of the back pack. These gave warnings for oxygen, water and carbon dioxide as well as for the suit’s pressure. On the front was a bracket upon which a crewman could attach his Hasselblad camera. Once the suit’s systems had been connected up, it was time for checks of the communications
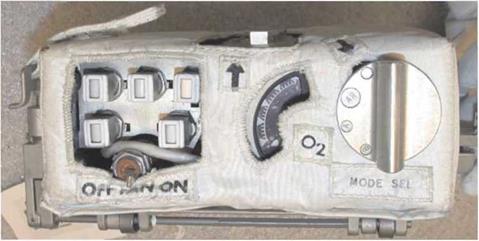
Remote control unit for the PLSS. (Training unit at the Smithsonian Institution’s Garber Facility, 2006. Photo courtesy Ulrich Lotzmann.)
|
between each other and with mission control. That’s when Conrad and Bean came unstuck.
The checklists the crews used were very thorough. Every connection and every switch position was detailed for the men to check, and the procedures were practised repeatedly before the mission. The problem for the Apollo 12 crew was that they had done this so many times that they thought they knew it. More importantly, they were used to their training hardware. A1 Bean explained: ‘’The gear used down at the Cape [for training] have the comm switches on them but you don’t have to use them at all for comm.” Hence they did not have to actually operate the switch during training, as Conrad pointed out, “fn training, we always just read it in the checklist and kept on going.”
“Here’s an example of the gear we’re using [on the Moon] not being configured precisely like the gear we use in practice; and that cost us,” recalled Bean. In fact it cost them nearly 20 minutes in a machine whose operational lifetime was measured in hours. Differences between training and flight hardware would bite them again when their TV camera was damaged by being inadvertently aimed at the Sun. The crew had only ever practised with an inert mock-up and had no appreciation of the flight unit’s susceptibility to direct sunlight.
With the final oxygen connections made from the OPS to the PLSS, each crewman took a last long drink from the LM’s water supply before they sealed themselves off by donning their helmets and gloves. Care was taken to ensure their microphones were properly positioned as there would be no opportunity to reposition them once outside. They then transferred the suit’s oxygen and cooling water hoses from the LM to the PLSS and put on their hard-wearing outer gloves. Because they would get no cooling from the PLSS until they were in a vacuum, crews from Apollo 12 onwards made sure to give themselves an extra shot of cooling water from the LM through their liquid-cooled garment before this changeover.
The next step was to make sure the leakage from their suits was acceptable. Rather than build suits that were completely airtight, engineers accepted a slight leak rate in the knowledge that the PLSS supply would be adequate to make up for the loss across the duration of the EVA. This ‘pressure integrity’ check required the suits to be inflated above cabin pressure until the gauges on their cuffs indicated 3.7
to four psi. The oxygen supply was then turned off and the gauge watched for a minute. Their checklist stipulated that they should not observe a drop greater than 0.3 psi, some of which was merely due to them breathing the oxygen or the gas getting into all the nooks and crannies within the suit.
Lost air
Once everyone was happy, it came time to let the air out of the cabin, or depressurise, to use the parlance. There were two valves the crew could use to achieve this; one on the forward hatch that led out to the ladder, and the other on the overhead hatch that would lead to the tunnel and the CSM once they redocked. The handles for these dump valves had three positions; open, closed and an intermediate position called ‘automatic’ which was its normal setting in which the valve acted to protect the spacecraft against excessive internal pressure. It remained closed until the cabin’s pressure reached a threshold of about one third of nonnal Earth atmospheric pressure with respect to the outside. It then opened to vent air until the pressure dropped below the threshold.
The procedure for depressurisation was to set the dump valve in its open position and drop the cabin pressure from its normal reading of about five psi until it indicated 3.5 psi and then stop. They then monitored their suits to ensure that they were also dropping to maintain the correct relative pressure. If all was well, the dump valve was returned to the open position until the cabin was evacuated enough that the forward hatch could be opened. When sensors indicated less than 3.5 psi, the EVA was deemed to have begun and Houston would begin to time the crew’s progress.
On Apollo 11, the depressurisation proved to be a lengthy procedure. Unlike subsequent missions, Eagle’s forward dump valve had been fitted with a bacterial filter which halved the rate at which air could depart the cabin. As the amount of air within the LM decreased, so did the pressure that was pushing it through the depress valve and thus the rate of evacuation tailed off. It took about three minutes for the cabin pressure to indicate only 0.2 psi but even this was too high for a crewman to directly open the hatch. It had to get nearer to 0.1 psi. Yet at such low pressures, the cabin began to be replenished by internal sources that further slowed the depressurisation, as Armstrong explained after the flight. “It took a very long time to depressurise the LM through the bacteria filter with the PLSS adding gases to the cockpit environment or something adding some cabin pressure. The second was that
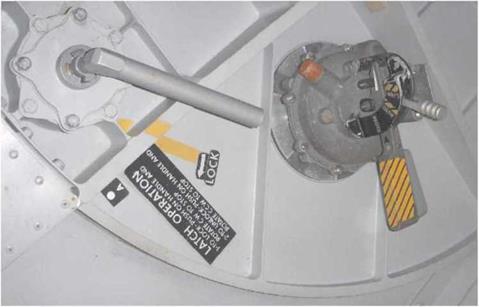
Detail of the overhead hatch with latch handle on the left and overhead dump valve on the right. (Courtesy, Frank O’Brien)
|
we weren’t familiar with how long it would take to start a sublimator in this condition. It seemed to take a very long time to get through this sequence of getting the cabin pressure down to the point where we could open the hatch, getting the water turned on in the PLSS, getting the ice cake to form in the sublimator, and getting the water alarm flag to clear so that we could continue. It seemed like it took us about a half hour to get through this depressurisation sequence.” To speed up the process on subsequent flights, not only was the filter dropped, crews took to grabbing the corner of the hatch and peeling it open.
The sublimator that Armstrong was referring to was the central element of the PLSS’s cooling system. Water was fed directly to the vacuum where its evaporation and eventual sublimation from ice to a gas cooled a separate water circuit that cooled the crewman. This emission of vapour was the reason that later crews precooled themselves before disconnecting their water from the LM. It was better not to start the sublimators until the hatch was open and a vacuum had been established in the LM.
Once the hatch was fully open, the commander manoeuvred himself onto the floor, kneeling with his feet towards the open hatch. Guided by his LMP, he crawled backwards through the hatch onto the porch, a small platform between the hatchway and the top of the ladder. When Neil Armstrong got onto the porch, he reached to his left and pulled a nearby D-ring which allowed one of the side panels of the descent stage to hinge open. A small TV camera was mounted upside down on the panel and strategically aimed to document his descent into the history books. On
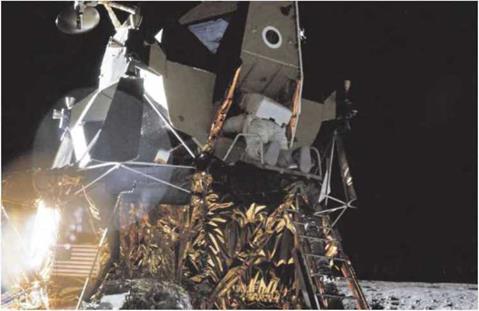
Alan Bean manoeuvring through Intrepid’& hatch. (NASA)
|
Earth, TV converters at the ground stations had circuitry to flip the image the right way up.
In the expectation that a descending LM was likely to deform the crushable interior of the landing legs and compress them, the lowest step of the ladder was set the best part of a metre above the footpad. However, on all missions, there was little appreciable compression of the gear, necessitating a gentle leap from the bottom step to the footpad in the low lunar gravity. Only then could a boot print be made in the lunar surface.