Dates: 16-24 July 1969
Crew: Commander – Neil Armstrong
Command Module Pilot – Mike Collins Lunar Module Pilot – Buzz Aldrin
Command Module Columbia Lunar Module Eagle
Lunar Touchdown: 20 July 1969, 4:10 PM EST Accomplishments: First manned landing on the Moon
J. L. Chen, How to Find the Apollo Landing Sites,
The Patrick Moore Practical Astronomy Series, DOI 10.1007/978-3-319-06456-7_3, © Springer International Publishing Switzerland 2014
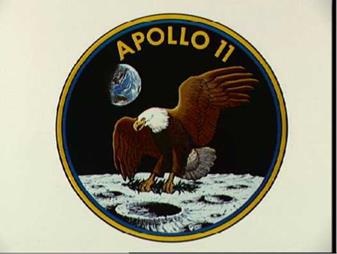
Fig. 3.1 Apollo 11. Courtesy of NASA
|
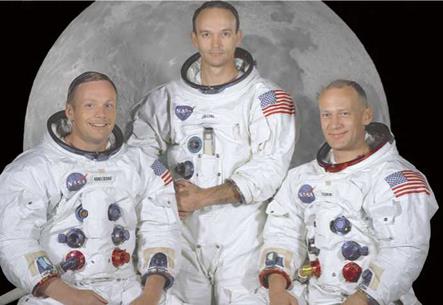
Fig. 3.2 Apollo 11 Insignia. Courtesy of NASA
|
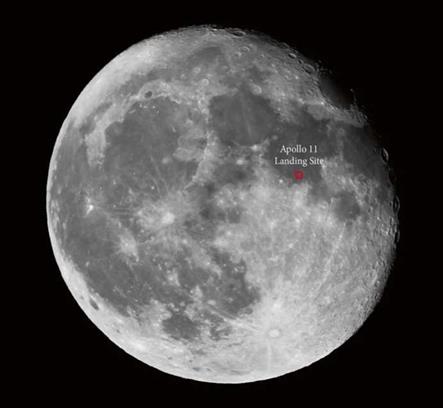
Fig. 3.3 Moon with Sea of Tranquility and Apollo 11 Landing Site. Courtesy of the author
|
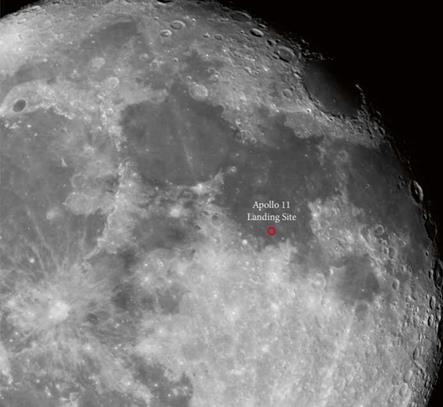
Fig. 3.4 First Zoom in on Apollo 11 Landing Site. Courtesy of the author
|
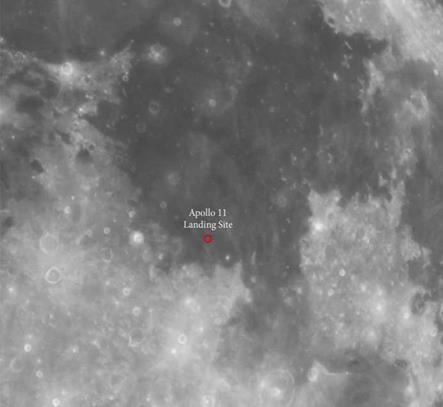
Fig. 3.5 Second Zoom in on the Apollo 11 Landing Site. Courtesy of the author. (Insert three zoom-in views indicating landing site)
|
The Apollo 11 Tranquility Base is probably the easiest to identify of all the Apollo landing sites. Mare Tranquillitatis, or the Sea of Tranquility as its commonly known, is in the middle of three roughly circular dark “Sea” areas that are strung together which an observer can see through binoculars or telescope. The Apollo 11 site is located near the southern end of the Sea of Tranquility, in a seemingly flat and clear area of the Moon. Binoculars or spotting scopes will enhance the view. Backyard astronomers with any size (60 mm aperture or greater) telescope can zoom in on the seemingly smooth region where Tranquility Base was established. Although the general area of the Apollo 11 landing zone can be appreciated by the backyard observer, the exact landing area is difficult to pinpoint because of the relatively featureless area chosen for the first landing. Three craters in the region of the landing site have been named for Armstrong, Aldrin, and Collins. These named craters are a challenge for the amateur astronomer, requiring a clear night with a steady atmosphere, 100 mm or greater aperture telescope, and moderately high power to resolve them.
The final landing site for Apollo 11 was selected for a myriad of conservative and safe reasons.
The Sea of Tranquility site was chosen because it had few craters and boulders, and a less than 2° slope in the approach path and landing site. Ironically, the actual mission required Neal Armstrong to fly the LM Eagle over a small crater filled with boulders. There were no large hills, high cliffs, or deep craters at the Tranquility site that could reflect erroneous altitude radar returns to the lunar module landing radar. It is readily apparent from a backyard telescope that the Sea of Tranquility represented a fairly featureless target area. The NASA mission planners tried to select a landing site that required the least expenditure of spacecraft fuel.
The actual landing required extensive maneuvering and resulted in the LM Eagle landing with only 20 seconds of fuel to spare. The Apollo 11 landing, as the first Moon landing, was risky and challenging. Five minutes into the descent burn, and 6,000 feet above the surface of the Moon, the LM navigation and guidance computer generated several unexpected 1201 (“Executive overflow – no vacant areas”) and a 1202 (“Executive overflow – no core sets”) alarms. The Guidance Controller, or GUIDO in NASA parlance, determined it was safe to continue the descent, and this was relayed to the crew. The executive overflows alarms meant the guidance computer could not complete all of its tasks in real time and had to postpone some of them. At the time, the cause for the alarms was diagnosed as the rendezvous radar switch being in the wrong position, which caused the computer to process data from both the rendezvous and landing radars at the same time. Software engineer Don Eyles concluded in a 2005 Guidance and Control Conference paper that the problem was actually due to a hardware design flaw, previously occurring once during testing of the first unmanned LM for Apollo 5. According to design and operational procedures, the rendezvous radar was warmed up in case of an emergency landing abort. The position of the rendezvous radar was encoded with synchros excited by a different timing reference source of 800 Hz AC than the one used by the computer. The two 800 Hz sources were frequency locked, but not phase locked, and small random phase variations made it appear as though the antenna was rapidly “dithering” in position even though it was completely stationary. These phantom shifts generated the rapid series of processing cycle “steals.” The extra spurious cycle stealing, as the rendezvous radar updated a counter, caused the computer alarms. The Apollo onboard flight software for both the CM and LM was developed using an asynchronous executive so that higher priority jobs could interrupt lower priority jobs. The sequence that occurred in the Apollo 11 landing was a successfully executed work around because of the software global error detection and recovery system. This included the restart capability to “kill and start over again”.
A little known fact about the 1201 and 1202 alarms during the final descent was their occurrence close to what NASA insiders referred to as the “Dead Man’s Zone.” Throughout the landing phase of the LM, an emergency abort could be accomplished by firing the ascent engine of the LM and returning to an orbital rendezvous with the CM. However, during the last 3 minutes of the landing approach, there was a 10 seconds block of time where the velocity of descent exceeded the ascent engine’s capability of returning the LM safely. A safe abort could be accomplished 10 seconds prior to or after the Dead Man’s Zone with no consequence. Attempting an abort during this Dead Man’s Zone would cause a total expenditure of the ascent stage fuel to just counteract the descent velocity, resulting in the LM running out of fuel and crashing to the surface of the Moon. The 1201 and 1202 alarms occurred within 20 seconds of the Eagle entering the Dead Man’s Zone. A split second decision by the NASA guidance controller Steve Bales, who knew the Eagle’s proximity to the Dead Man’s Zone, and recognized and ignored the alarms resulted in a successful Apollo 11 landing. Bales was honored after the mission to accept a NASA Group Achievement Award from President Richard Nixon on behalf of the entire mission operations team.
With the recent passing of Neil Armstrong, there has been a discussion about his famous “That’s one small step for Man, one giant leap for Mankind”. For years, Neil Armstrong claimed that his first words included the word “a”, as in “…one small step for a Man.”, especially since the quoted line actually doesn’t make any sense. In 2006, Australian computer expert Peter Shann Ford conducted a computer sound analysis of the radio transmission of Armstrong’s first words from the Moon. The analysis indicated an acoustic wave from the word “a” spoken by Armstrong at a rate of 35 milliseconds. The word “a” was spoken too fast for it to be audible in the transmission, but the analysis confirms that Armstrong actually spoke the line as he had planned.
As an aside, the one small step was not so small. Neil Armstrong’s precise piloting produced a landing that was so gentle, the LM’s shock absorbers did not compress. Armstrong’s one small step was a leap of 3.5 feet!
Little known by the general public, the original video and telemetry captured by NASA during the first lunar EVAs has been lost. Over the years, the Slow-Scan Television (SSTV) video data tapes of the first steps on the Moon have either been lost, misplaced, or accidentally destroyed. The late 1960s state-of-the-art required a live video signal from the Moon to be 10 frames per second at 320 lines of resolution, hardly high-definition television of today. This video signal was multiplexed in with the telemetry data and transmitted to Earth. In those years, NASA routinely erased and re-used data tapes due to the high cost of the tapes and lack of an alternative archiving medium. NASA made efforts in 2006 to locate any surviving tapes, with some telemetry data tapes with no video being located. What remains are the now-famous low resolution, grainy videos that the television networks captured at the time. It is felt that if the original tapes are ever found, modern up-conversion techniques would yield improved video images.
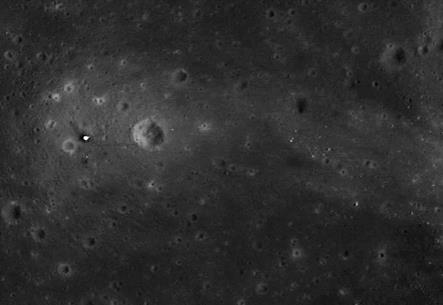
Fig. 3.6 Lunar Reconnaissance Orbiter view of the Apollo 11 Landing Site. Photo courtesy of NASA and Arizona State University
|
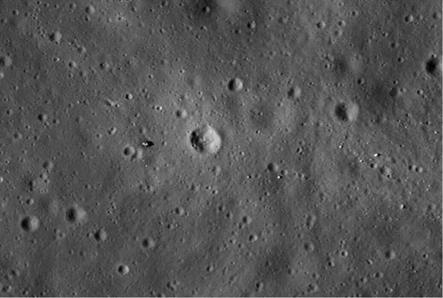
Fig. 3.7 LRO view of Apollo 11 Landing site. The Lunar Ranging Retro Reflector (LRRR) and the Passive Seismic Experiment (PSE) are identifiable in this photo. Photo courtesy of NASA and Arizona State University
|
The distance to the Moon can be accurately determined by firing an earthbound laser off one of the LRRR’s, left behind by Apollo 11, 14, and 15. The information is used to study lunar recession due to Moon’s interaction with the Earth. The LRRRs are the only experiments currently used today. The PSE was used to provide data on moonquakes and other disturbances to study the Moon’s subsurface structure.
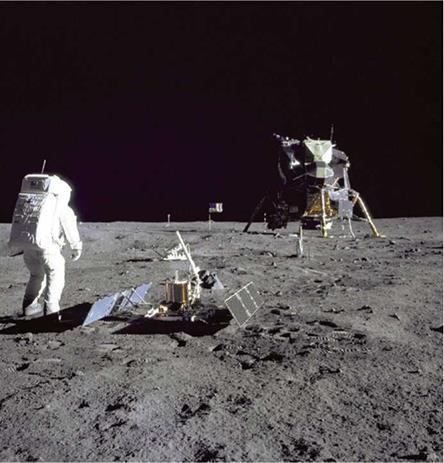
Fig. 3.8 Mission picture of Astronaut Buzz Aldrin during the Apollo 11 extravehicular activity on the Moon. He is standing near the Early Apollo Scientific Experiments Package (EASEP). Shown in the foreground is the Passive Seismic Experiment (PSE); beyond is the Laser Ranging Retro-Reflector (LRRR). Photo courtesy of NASA
|
The EASEP was replaced in later missions with the Apollo Lunar Surface Experiments Package (ALSEP). The EASEP was a very limited science package, primarily because of the limited time allotted for the extravehicular activities of the Apollo 11 crew. The PSE, along with similar seismic monitors of the ALSEP of later Apollo missions, continued collecting data and relaying the information to NASA until 1977, when all six seismic experiments were turned off. To this day, the Apollo 11 LRRR, along with identical LRRRs deployed by the subsequent Apollo missions, remains as the only experiment still active. The LRRR is a passive experiment consisting of a series of corner-cube reflectors. Corner-cube reflectors are a special type of mirror with the property of always reflecting an incoming light beam back in the originating direction. Earthbound lasers aimed at the LRRR receive the reflected laser return to precisely determine the distance between the Earth and the Moon. Over the years of conducting precise measurement data using the Apollo LRRRs, scientists have refined the knowledge base as follows:
1. Improved definition of the Moon’s orbit.
2. The rate the Moon is receding from the Earth is 3.8 cm per year.
3. Discovered variations in the Moon’s rotation, with implications on the size of the Moon’s core.
4. Improved and refined the Earth’s rotational changes, and has given more precision to the precession of Earth’s spin axis.
5. Useful in providing additional proof of Einstein’s Theory of Relativity.
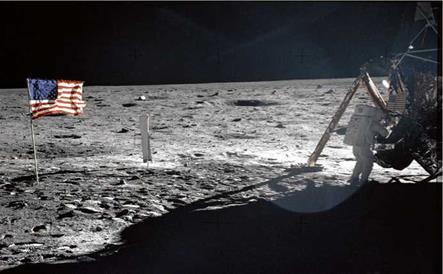
Fig. 3.9 Astronaut Neil Armstrong, at the modular equipment storage assembly (MESA) of the Lunar Module Eagle, on the historic first moon walk on the lunar surface. Interestingly, this is one of the few photographs that shows Neil Armstrong during the Apollo 11 mission’s time on the Moon. Photo courtesy of NASA
|
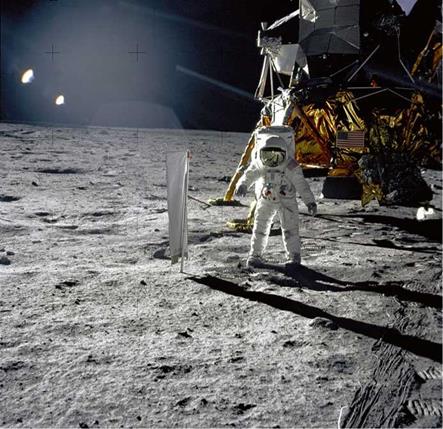
Fig. 3.10 Here, astronaut Buzz Aldrin is photographed next to the Lunar Module “Eagle.” On Aldrin’s right is the Solar Wind Composition (SWC) experiment. Photo courtesy of NASA
|
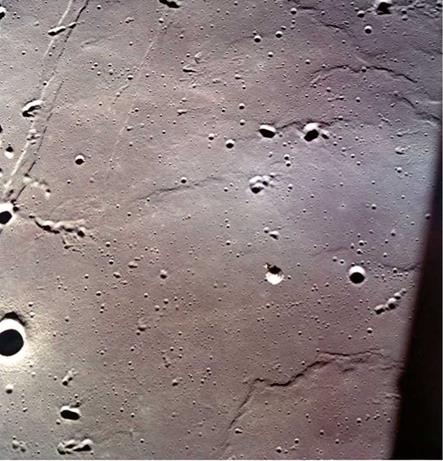
Fig. 3.11 Tranquility Base as seen from the Apollo 11 Command Module, taken by Astronaut Mike Collins. This is an opportunity to compare and contrast this photo taken in 1969, and the more recent LRO photos of the same area. Ironically, although Collins took this picture, he never visually sighted the LM Eagle on the surface from the orbiting CM. Photo courtesy of NASA
|
(Outward/Inward)
An interesting aside to the Apollo 11 mission was revealed many years later. Apparently, the U. S. Customs required the Apollo 11 crew to fill out a declaration form for the moon rocks and lunar samples returned from the Sea of Tranquility. As seen in Fig. 3.12, the Customs declaration form is signed by Astronauts Armstrong, Aldrin, and Collins, declaring moon rocks and moon dust samples, and their destination was the Moon.
Apollo 11 collected the first geologic samples from the Moon. The first task of the mission was for Neil Armstrong to take a lunar soil sample and place it in his space – suit pocket, such was the high priority placed in gathering lunar soil samples. This contingency lunar surface sample was made in the first 3.5 minutes in the case an emergency liftoff was required and the mission was cut short. Astronauts Armstrong and Aldrin eventually collected almost 50 pounds of lunar material, including 50 rocks, samples of the fine-grained lunar soil, and two core tubes that included material from up to 13 cm below the Moon’s surface. The Apollo 11 samples contained no water and provided no evidence for life at any time in the Moon’s history. Two main types of rocks, basalts and breccias, were found at the Apollo 11 landing site.
On Earth, basalts are a common type of volcanic rock. Basalts are generally dark gray in color; when one looks at the Moon in the night sky, the dark areas are basalt. The basalts found at the Apollo 11 landing site are generally similar to basalts on Earth and are primarily of the minerals pyroxene and plagioclase. The basalt samples returned by Apollo 11 contain more of the element titanium than is usually found in basalts on Earth. The basalts returned by Apollo 11 range in age from 3.6 to 3.9 billion years, which scientists believe are from at least two chemically different magma sources.
The Moon has been bombarded by a countless number of meteorites over the eons. These impacts have broken many rocks up into small fragments. The heat and pressure of such impacts sometimes fuses small rock fragments into new rocks, called breccias. The rock fragments in a breccia can include both mare basalts as well as material from the lunar highlands. The lunar highlands comprise primarily of a light-colored rock known as anorthosite, which consists primarily of the mineral plagioclase. It is very rare to find rocks on Earth that are virtually pure plagio – clase. On the Moon, it is believed that the anorthosite layer in the highland crust formed very early in the Moon’s history when much of the Moon’s outer layers were molten. This stage in lunar history is known as the magma ocean. The plagioclase – rich anorthosite floated on the magma ocean like icebergs in the Earth’s oceans.
Three previously unknown minerals were discovered in the lunar samples of Tranquility Base:
Armalcolite is a titanium-rich mineral first discovered in the Apollo 11 samples, and later appearing samples from the Taurus-Littrow Valley and the Descartes Highlands. This newly discovered lunar mineral was appropriately named after the Apollo 11 astronauts: ARMstrong, ALdrin, and COLlins. Armalcolite is a minor mineral found in basalt rocks high in titanium content. Armalcolite has been identified on Earth, initially from samples taken from Smokey Butte, Garfield County, Montana. Scientists have also been able to synthesize Armalcolite in the laboratory, by using low pressures, high temperatures and a rapid quenching from about 1,000 °C to the ambient temperature.
Tranquillityite is a silicate mineral first discovered in Apollo 11 rock sample 10,047. Obviously taking its name from the site of the Apollo 11 landing, Tranquillityite has been found in rock samples from all the Apollo missions. It was long considered the only mineral found solely on the Moon, until 2011, when samples taken from six regions in the Pilbara region of Western Australia were found to contain this mineral.
Pyroxferroite is the third of the family of minerals discovered from the Apollo 11 lunar samples, . Pyroxferroite has since been discovered in meteorites of lunar and Martian origin. Terrestrially, the mineral has been discovered in Kyoto Prefecture, Japan, in Vaarmland, Sweden, in Lapua, Finland, and here in the U. S. in Anderson County, South Carolina. Like Armalcolite, Pyroxferroite can also be produced in the laboratory by annealing synthetic clinopyroxene at high pressures and temperatures.
Following the historic Apollo 11 mission, the Moon rocks and soil samples were distributed to over 150 scientific laboratories worldwide. In at least one case, 20 vials of moon dust were misplaced and lost for over 40 years. In May, 2013, an archivist uncovered vials of Apollo 11 moon dust while tidying up a storage space at Lawrence Berkeley National Laboratory in California. The samples, with handwritten labels marked 24 July 1970, were originally delivered to the Space Sciences Laboratory in Latimer Hall on the University of California-Berkeley campus. After the experiments were completed, the samples procedurally should have been returned to NASA. A mystery to all, the vials of lunar dust ended up in storage until the recent discovery.
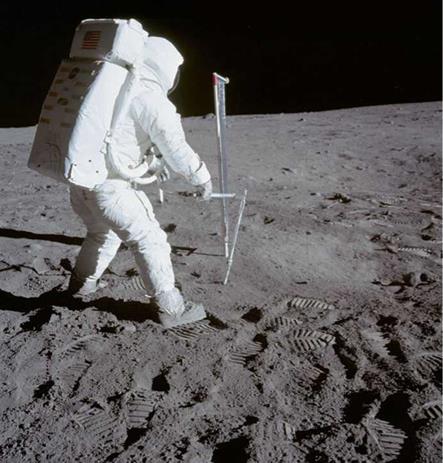
Fig. 3.13 Aldrin deploying the Solar Wind Composition Experiment. Photo courtesy of NASA
|
As part of every Apollo mission, a solar wind composition experiment was deployed in order to collect samples of the solar wind. The astronauts deployed 1.4 m x 0.3 m sheet of aluminum foil mounted on a five section telescoping pole, which exposed collected particles from the solar wind. The foil was gathered up and stored in the LM Eagle prior to lunar liftoff to be brought back to Earth for analysis. The Apollo 11 foil was exposed for 77 minutes. Subsequent missions allowed for longer exposures, culminating with the Apollo 16 exposure of 45 hours. The results of the Apollo 11 solar wind composition experiment measured embedded light noble gases, such as He-3, He-4, Ne-20, Ne-21, Ne-22, and Ar-36, and found the variations in solar wind composition. These variations correlated with variations in the intensity of the solar wind as determined from magnetic field measurements.
Armstrong and Aldrin’s departure from the Moon, although not as dramatic as the landing, was not without missteps. The astronauts planned to place mementos on the surface of the Moon, including a mission patch for the ill-fated Apollo 1 to honor their comrades Gus Grissom, Ed White, and Roger Chaffee. Also to be left behind were medals commemorating Soviet cosmonauts Vladimir Komarov and Yuri Gagarin who died in flight in 1967 and 1968. The goodwill messages of 73 world leaders and a small gold olive branch pin completed the package. However, the schedule for lunar surface activities had left little time for ceremony, resulting in Aldrin unceremoniously dumping the contents of his spacesuit pocket onto the Moon surface prior to boarding the LM Eagle for the last time. Upon re-entering the LM and preparing for the launch, the astronauts accidentally broke the switch responsible for igniting the ascent engine, potentially threatening to strand them on the Moon. Oops! Aldrin used a pen to toggle the switch, thus avoiding being marooned on the Moon. On liftoff from the Moon, the item which gave the astronauts the most problems during setup toppled over. Seen prominently in videos and photographs from the mission, the American flag proved to be the most troublesome mission item to setup. The Moon surface proved to be harder than anticipated, and the flag pole was not securely implanted into the Moon’s surface. On liftoff, Aldrin reported seeing the Sears-made American flag topple over from the ascent engine’s exhaust.
Rendezvous with the Columbia CM had problems too. During the process of rendezvous and docking the Eagle to Columbia, the LM inadvertently reached gimbal lock, causing the spacecraft inertial guidance systems to lose orientation alignment in space and tumble briefly. Armstrong and Aldrin used the abort guidance system for attitude control and quickly recovered.
To top off the publicly-viewed “perfect” mission, after re-entry and landing in the Pacific Ocean, the Columbia landed in upside down “Stable 2” position. As designed, the inflatable air bags righted the spacecraft.
To the public, the Apollo 11 mission seemed flawless. In the bright light of reality, the crew and the Mission Control team were able to work around a number problems and made the mission a success.
In July 2013, an expedition funded and headed by Amazon founder and CEO Jeff Bezos recovered parts of at least two Saturn V F-1 booster engines from the
Atlantic Ocean. Using state-of-the-art sonar technology and tethered remote controlled underwater vehicles, a team of underwater archeologists, conservators, and underwater salvage experts were able to locate, map, catalog, and recover the F-1 engine components. Examination of the recovered thrust chamber revealed the number 2044, which was the serial number given by the F-1 manufacturer Rocketdyne. This number correlated to the NASA assigned number 6044, which was the number five F-1 engine of the Apollo 11 Saturn V launch vehicle. This discovery positively identified the final resting place of the first stage of the Saturn V that hurled Armstrong, Aldrin, and Collins into space in their historic journey to the Moon.