By the end of the Second World War, aviation was already well into the jet age, and man was flying yet higher and faster in his quest for space. During the years after the end of the war, human factors research continued to evolve in support of this movement. A multiplicity of human and animal studies were conducted during this period by military, civilian, and Government researchers to learn more about such problems as acceleration and deceleration, emergency egress from high-speed jet aircraft, explosive decompression, pressurization of suits and cockpits,
and the biological effects of various types of cosmic rays. In addition, a significant amount of work concentrated on instrument design and cockpit display.[327]
During the years leading up to America’s space program, humans were already operating at the edge of space. This was made possible in large part by the cutting-edge performance of the NACA-NASA highspeed, high-altitude rocket "X-planes”—progressing from the Bell X-1, in which Chuck Yeager became the first person to officially break the sound barrier, on October 14, 1947, to the phenomenal hypersonic X-15 rocket plane, which introduced man to true space flight.[328]
These unique experimental rocket-propelled aircraft, developed and flown from 1946 through 1968, were instrumental in helping scientists understand how best to sustain human life during high-speed, high-altitude flight.[329] One of the more important human factors developments employed in the first of this series, the Bell X-1 rocket plane, was the T-1 partial pressure suit designed by Dr. James Henry of the University of Southern California and produced by the David Clark Company.[330] This suit proved its worth during an August 25, 1949, test flight, when X-1 pilot Maj. Frank K. "Pete” Everest lost cabin pressure at an altitude of more than 65,000 feet. His pressure suit automatically inflated, and though it constricted him almost to the point of incapacitation, it nevertheless kept him alive until he could descend. He thus became the first pilot saved by the emergency use of a pressure suit.[331]
During the 1950s and 1960s, the NACA and NASA tested several additional experimental rocket planes after the X-1 series; however, the most famous and accomplished of these by far was the North American X-15. During the 199 flights this phenomenal rocket plane made from 1959 to 1968, it carried its pilots to unprecedented hypersonic speeds of nearly 7 times the speed of sound (4,520 mph) and as high as 67 miles above the Earth.[332] The wealth of information these flights continued to produce, nearly right up until the first piloted Moon flight, enabled technology vital to the success of the NASA piloted space program.
One of the X-15 program’s more important challenges was how to keep its pilots alive and functioning in a craft traveling through space at hypersonic speeds. The solution was the development of a full – pressure suit capable of sustaining its occupant in the vacuum of space yet allowing him sufficient mobility to perform his duties. This innovation was an absolute must before human space flight could occur.
The MC-2 full-pressure suit provided by the David Clark Co. met these requirements, and more.[333] The suit in its later forms, the A/P-22S-2 and A/P-22S-6, not only provided life-sustaining atmospheric pressure, breathable oxygen, temperature control, and ventilation, but also a parachute harness, communications system, electrical leads for physiological monitoring, and an antifogging system for the visor. Even with all these features, the pilot still had enough mobility to function inside the aircraft. By combining the properties of this pressure suit with those of the X-15 ejection seat, the pilot at least had a chance for emergency escape from the aircraft. This suit was so successful that it was also adapted for use in high-altitude military aircraft, and it served as the template for the suit developed by B. F. Goodrich for the Mercury and Gemini piloted space programs.[334]
The development of a practical spacesuit was not the only human factors contribution of the X-15 program. Its pioneering emphasis on the physiological monitoring of the pilot also formed the basis of that used in the piloted space program. These in-flight measurements and later analysis were an important aspect of each X-15 flight. The aero – medical data collected included heart and respiratory rates, electrocardiograph, skin temperature, oxygen flow, suit pressure, and blood pressure.
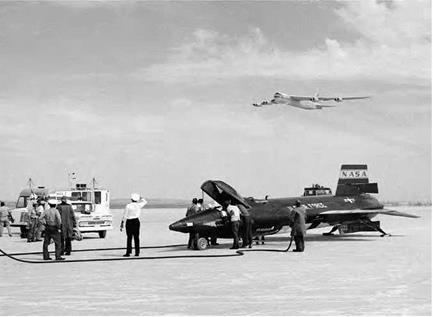
The X-15 on lakebed with B-52 mother ship flying overhead. Lessons learned from this phenomenal rocket plane helped launch humans into space. NASA.
|
Through this information, researchers were able to better understand human adaptation to hypersonic high-altitude flight.[335]
The many lessons learned from these high-performance rocket planes were invaluable in transforming space flight into reality. From a human factors standpoint, these flights provided the necessary testbed for ushering humans into the deadly environment of high-altitude, high-speed flight—and ultimately, into space.
Another hazardous type of human research activity conducted after World War II that contributed to piloted space operations was the series of U. S. military piloted high-altitude balloon flights conducted in the 1950s and 1960s. Most significant among these were the U. S. Navy Strato – Lab flights and the Air Force Manhigh and Excelsior programs.[336]
The information these flights provided paved the way for the design of space capsules and astronaut pressure suits, and they gained important biomedical and astronomical data.
The Excelsior program, in particular, studied the problem of emergency egress high in the stratosphere. During the flight of August 16, 1960, Air Force pilot Joseph Kittinger, Jr., ascended in Excelsior III to an altitude of 102,800 feet before parachuting to Earth. During this highest-ever jump, Kittinger went into a freefall for a record 4 minutes 36 seconds and attained a record speed for a falling human body outside of an aircraft of 614 mph.[337] Although, thankfully, no astronaut has had to repeat this performance, Kittinger showed how it could be done.
Yet another human research contribution from this period that proved to be of great value to the piloted space program was the series of impact deceleration tests conducted by U. S. Air Force physician Lt. Col. John P. Stapp. While strapped to a rocket-propelled research sled on a 3,500-foot track at Holloman Air Force Base (AFB), NM, Stapp made 29 sled rides during the years of 1947-1954. During these, he attained speeds of up to 632 mph, making him—at least in the eyes of the press— the fastest man on Earth, and he withstood impact deceleration forces of as high as 46 times the force of gravity. To say this work was hazardous would be an understatement. While conducting this research, Stapp suffered broken bones, concussions, bruises, retinal hemorrhages, and even temporary blindness. But the knowledge he gained about the effects of acceleration and deceleration forces was invaluable in delineating the human limitations that astronauts would have while exiting and reentering the Earth’s atmosphere.[338]
All of these flying and research endeavors involved great danger for the humans directly involved in them. Injuries and fatalities did occur, but such was the dedication of pioneers such as Stapp and the pilots of these trailblazing aircraft. The knowledge they gained by putting their lives on the line—knowledge that could have been acquired in no other way—would be essential to the establishment of the piloted space program, looming just over the horizon.