Guidance and navigation underpinned much of the challenge of Apollo. Indeed, it is crucial to any form of spaceflight and, consequently, major systems on board the command module and lunar module were devoted to it, as were a large number of consoles in the mission operations control room (MOCR, pronounced to rhyme with ’poker’). The front row of the MOCR, known to its occupants as The Trench’, was where the flight dynamics guys sat, and on a single shift two flight controllers, GNC (guidance, navigation and control) for the command module and Control for the lunar module, kept a close watch on the hardware with which guidance and navigation was performed. A further three – the guidance officer (Guido), the flight dynamics officer (FIDO) and the retrofire officer (Retro) – thought about nothing other than where the spacecraft was, where it was going and how it could return to
W. D. Woods, How Apollo Flew to the Moon, Springer Praxis Books,
DOI 10.1007/978-1-4419-7179-1 6. © Springer Science+Business Media. LLC 2011
Earth if something went wrong, respectively. As this is a necessarily complex topic that is not always amenable to verbal description, and one that requires a certain amount of three-dimensional visualisation, it is worth taking some time out to discuss the problem and consider how it might be solved.
Guidance is how to make a spacecraft go where intended. Given its constraints in propellant and payload, what path does a spacecraft need to take to get from one place to another?
Navigation is how to determine where a spacecraft is. How can its position and velocity be determined at any given time as it passes between worlds?
Control is the operation of the hardware to ensure that a spacecraft reaches its intended destination. Contrary to a misconception raised by the entertainment industry, spacecraft do not fly around the cosmos with their engines ablaze; with the exception of recent craft whose ion engines have thrust levels measured in millinewtons. Instead, everything in space, be it spacecraft, our Earth or the entire galaxy, generally coasts along following ballistic paths, moving in freefall with their motion determined by gravity. A major step towards guiding a spacecraft is to understand how those paths work, and how they might be measured, predicted and then controlled.
At its simplest, Apollo’s path to the Moon can be likened to throwing a stone. If you throw a stone almost vertically, it follows a sharply curved path which travels
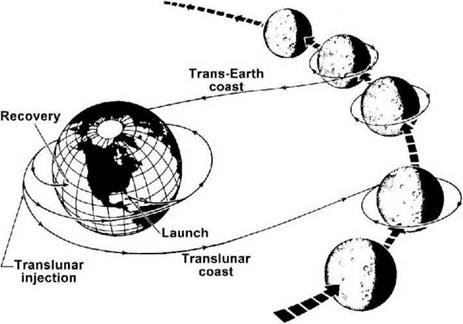
Diagram of Apollo’s path to and from the Moon. (Simplified from the Apollo 8 Press Kit^NASA)
|
most slowly when it is near the top of that path. Apollo’s trajectory was directly comparable. After the spacecraft had entered Earth orbit, it was ‘thrown’ towards the Moon by the S-IVB stage on an extended, curved trajectory away from Earth, governed by the same natural laws that took your stone along its ballistic arc; one that, in the absence of intervention, would reach a slow peak before falling back to Earth.
The trick for lunar flight was to work out the time and duration of the S-IVB’s throw that would cause the Moon’s gravity to intervene on the spacecraft’s path in a beneficial manner. By careful calculation, NASA’s trajectory experts picked the correct place and time, and just the right amount of shove from the S-IVB’s engine, to set up a rendezvous between the spacecraft and the Moon. For the three days during which Apollo coasted on its extended ellipse out from Earth, the Moon moved a quarter of a million kilometres along its own orbit (about one-tenth of a complete revolution).
Had the technology of spaceflight produced some fabulous ship with unlimited propellant and great power, the trip could have been made much more quickly by having the crew fire their engines all the way there and back, using brute force to expedite the journey. Unfortunately, no space faring nation has yet had that luxury as even ion engines must operate for weeks or months for their low thrust to have an appreciable effect. Lifting propellant off Earth’s surface and into space is an extremely expensive proposition. Having paid dearly to get it there, it must be used in the most efficient manner possible in order to avoid carrying any more propellant than is needed for the task. It was this type of thinking that allowed the Apollo missions to be accomplished with a single Saturn V in the first place. Until the advent of low-thrust ion engines that operate for months on end, most space flight was
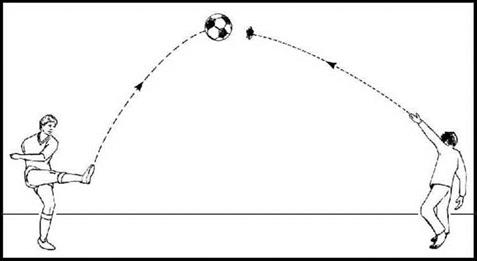
Diagram of a stone and football thought experiment.
|
conducted with chemical rockets that used short engine burns to modify long coasting nights.
It is helpful to think of the problem in terms of our stone-throwing analogy. Imagine that someone kicks a football high across a field and that you throw your stone from underneath, at the right time with the right force to hit the ball. This would be analogous to a spacecraft being sent to the Moon and impacting its surface – not something Apollo would want to do. but a fate that deliberately befell the Ranger probes in the early-to-mid-1960s. But if the stone had been travelling a little more slowly, the football would fly across its path early, leaving the stone to coast past the ball’s trailing side. If the stone had been moving a little faster, it would reach the football’s flight path before the football arrived, and would therefore pass in front of it. finally, if there was a way to control the timing and force of the throw accurately, and to monitor the stone’s flight path very carefully and change it if required, then the stone could be made to pass the leading side of the football by any number of millimetres we wished.
Getting an Apollo spacecraft to the Moon was a very similar exercise except that a football has no appreciable gravity and the Moon docs, and this had to be taken into account. If the trajectory produced by the ТІЛ burn was correct, the spacecraft would be pulled around the lunar far side, miss the Moon by about 100 kilometres, and be slung in the direction of Earth in an approximation of the intrinsically safe free-return trajectory. Midway around the far side was when the spacecraft had to intervene by firing its SPS engine. This would be done near the point of closest approach, against the direction of travel in order to slow’ the spacecraft and enter lunar orbit. But what would happen if the Moon-bound trajectory was not precisely right? If the spacecraft were moving towards the Moon too quickly, it would reach the Moon’s distance too early and pass over the surface at a higher altitude. A failure of the SPS engine would leave it to sail on into deep space, never to return, at least not with its crew still alive. At the other end of the error scale, an ever-so-slightly slow coast would take them closer to the Moon, creating a very real risk of the spacecraft impacting the lunar surface as it swung around the far side.
It was obviously critical to the lives of the crew1 that the spacecraft be placed onto the ideal Moon-bound trajectory. It was equally important to determine whether or not that trajectory was being followed and if not, to do something about it. One of the inherent problems in ballistic flight is that tiny perturbations early in a trajectory have large effects when propagated forward to a destination. Even an apparently perfect trajectory from the ТІЛ burn contained errors, initially too small to measure, but whose effects became apparent through time. Additionally, ground controllers had to understand the many factors that could interfere with Apollo’s trajectory, the most significant of which was the size and direction of the push given to the spacecraft by the S-IVB at translunar injection. Despite being well-engineered and controlled, this rocket stage, like any rocket in existence, was unlikely to deliver a perfect burn. There was always some small deviation from the ideal that would later have to be compensated for. Additionally, as the spacecraft coasted to its destination, housekeeping manoeuvres carried out by the crew using the RCS thrusters tended to affect the trajectory. Also, the expulsion of any liquids or gases
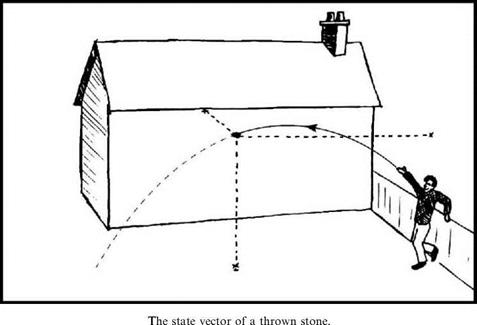
|
by the crew as part of their daily operation generated tiny thrust forces. Water vapour from the spacecraft’s cooling system, waste water from the fuel cells as they generated electricity, and urine from the crew were all necessary emissions that generated sufficient thrust to deflect the trajectory. To compensate for all these compounded perturbations, the crew had to make small correction burns. However, they had to know how much correction to make, and to do this it was necessary to measure their trajectory with extreme accuracy.