The LM approached the CSM with its windows facing its quarry. When originally envisaged, the LM was to have had two docking ports, the second being at the forward hatch. But in the drive to cut weight from the spacecraft, the heavy docking collar was replaced by a simple square hatch through which a suited crewman and his back pack could crawl on his way to the lunar surface. With the docking port at the top of the ascent stage, a small window was installed above the commander’s head to enable him to view through the roof of the LM. Having lined up in front of the CSM, he had to pitch down until the docking apparatus of both spacecraft faced each other, essentially lining up their v axes. The LM was then rotated 60 degrees to
-Y
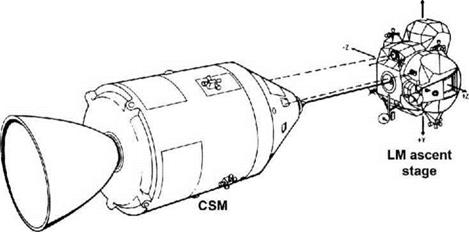
Docking alignment of the CSM and the LM ascent stage. (NASA)
|
line up the docking aids between the two spacecraft. From here, the CMP took over m bring the spacecraft together and dock. The commander could carry out the docking, but to do so would have meant craning his head backwards uncomfortably he was still fully suited. It was much easier for the commander to hold the LM steady while the CMP, who was comfortably viewing through a rendezvous window, brought the CSM up to the small spacecraft.
When Armstrong was manoeuvring Eagle for docking, he decided to change the procedure, but soon wished he hadn’t. He realised that if he were to pitch down at that point, the Sun would shine straight into his eyes. Therefore, he chose to line up 60 degrees further around before he pitched down, thereby avoiding the Sun. Having done so, Collins asked him to rotate a little further to get the docking aids properly aligned.
“We complied and promptly manoeuvred the vehicle directly in the gimbal lock.” related Armstrong in the debrief. Having gone into gimbal lock, the PGNS was no longer able to hold the vehicle stable. "I wasn’t aware of it because I was looking out the top window. No doubt, we were firmly ensconced in gimbal lock. We had all the lights on.” Bui they had a backup system. “We just pul il in AGS and completed the docking in AGS. This was just a goof on our part. We never should have arrived at the conclusion from any series of manoeuvres. However, that’s how ii happened. Ii wasn’t significant in this ease, bui il certainly is never a desirable thing to do.”
Unfortunately, holding the LM siable using the AGS had a side effect that caught Collins unaware once he had soft docked and began to retract the docking probe for a hard dock. As the probe began to pull the light ascent stage towards the much heavier CSM, ihe AGS delected a change in altitude and furiously tried to compensate for it by firing the LM’s thrusters. Collins didn’t realise this and began his own aitcmpi to correct ihe motions of the spacecraft relative to one another, but as the two spacecraft were flexibly joined at the capture latches, their motions were somewhat complex. "That was a funny one.” he remarked immediately afterwards. “I thought things were pretty steady. I went to ‘retract’ there, and that’s when all hell broke loose. [The LM was] jerking around quite a bit during the retract cycle.” In eight seconds the time it took the probe to retract the problem disappeared as the two spacecraft became one. But this incident worried engineers who looked into the dynamical issues of having two vehicles joined by a flexible probe.
Dick Gordon and Pete Conrad had no such worries bringing CSM Yankee Clipper and LM Intrepid together on Apollo 12. “It was a simple, easy task to perform,” said Gordon post-flight. “It could have been done in darkness as well as daylight with just as much ease. I don’t think that the vehicles moved hardly at all at contact. There was certainly no noticeable motion, anyway.”
Conrad concurred: “We came right in and stopped. I pitched over and did the yaw manoeuvre after Dick did his roll. We did the docking just the way we stated before. Dick came in and docked; I maintained attitude hold – tight dead band. As soon as he got his top latches barber poled, we went to Free. Neither spacecraft so much as moved a muscle, and we got a complete, good lock. He straightened out
attitude with his translations thrusters and went to hard dock, and it pulled us right in there without either spacecraft deviating; bango! We had 12 latches.”
When Eugene Cernan brought Apollo 17’s ascent stage. Challenger, to rendezvous with the CSM America he thought the spacecraft was a fine sight to see.
“Okay. I’ve got you right out the overhead, Ron." called Cernan to his CMP Ron Evans. He had pitched over to face the LMs drogue at the CSM’s docking probe and the mirror-like surface of the command module looked resplendent in the sunshine. “Now I’m going to yaw.”
“Okay, yaw her around,” replied Evans as Cernan began to line up the two craft to get the docking aids aligned.
“Okay, here we go.” Cernan was enjoying the responsive spacecraft he had under his fingertips. “What a super flying machine!”
“Still looks kind of tinny to me.” mocked Evans.
“Command module looks just as good as the day they pul it on the pad,” said Cernan.
“And, you know-, so does Challenger, by gosh.” said Evans. “You’re missing some of the pieces.” The last time he had seen Challenger, it had a descent stage attached, one that still sits quietly on the Moon.
“Yes, one big piece we left behind.”
When Evans tried to dock with Challenger, he found the lightness of the LM took a bil of getting used to. His approach speed was only two or three millimetres per second. “Coming in nice and slow; no problems,” he informed his commander.
“Okay, you’re looking good, babe.” said Cernan encouragingly. “I got you on my COAS right up in the middle of the window. Looking good. Must be a couple of feet away.”
“Stand by,” warned Evans as he neared the point where 16.3 tonnes of CSM would impact 2.3 tonnes of LM ascent stage whose tanks were nearly empty. But the capture latches failed to engage with the inside lip of the drogue. Evans had brought the CSM in too slowly.
“Okay; I didn’t get it. Let me plus-.v it.”
“Okay. You didn’t get it," confirmed Cernan.
“Might have been a little bit slow. Stand by.” Evans went to have another go but this time driving the probe home by firing his thrusters to give the CSM a positive push in the plus-.v direction.
“You got it! Capture!” Cernan had heard the three latches at the tip of the probe engage.
“Barber pole,” called Evans, as he saw the ’talkback’ indicators on his instrument panel change to show that they had latched. "Capture, go Free.”
Evans’s call for Cernan to ’go Free’ meant that he wanted the LM to stop trying to hold its attitude. The lightweight spacecraft was at the end of the probe and any motion it had would be damped out by the probe’s articulated tip.
“Crazy thing,” muttered Evans as he waited for the CSM and LM to line up on the end of the probe.
Cernan wondered what the problem was: "Say again?”
"I get the right…” Evans laughed at the jittery LM. “And then it goes around the other way. I think you’re bouneing around up there, too, you know.”
”J know it. I’m just swinging free,” replied Cernan.
“You’re bouncing around more on the probe.” said Evans. “See, I’m not moving at all.”
To try to stabilise the situation. Evans suggested that Cernan allow the LM to hold its attitude again. Thruster jets began to pull the small spacecraft onto the attitude it had been programmed to keep. As it did so. it also applied a small torque to the CSM via the probe.
“Okay. I’m stable now,’’ called Cernan once the LM’s motions had damped out. “Okay. Now let me come up to you."
“Okay, when you’re happy. I’ll go free. Looking good now.”
“Looking good, yes.” confirmed Evans. “See that’s what we needed. Okay. Why don’t you go to Free, and we’ll go to retract?"
“Okay,” said Cernan. “I’m Free.” Cernan once more stopped the LM from controlling its attitude.
“Okay, retract. Here you come.” The struts of the probe mechanism began to fold, in the process drawing the two halves of the docking tunnel together. “Bang! I got two barber poles.”
“You got what?” quizzed Cernan.
“Okay,” laughed Evans. lie had got the sense of the talkback indicators the wrong way round. "Two greys, I mean.”
Cernan shared the humour. "That’s better. Sounded good in here.’’
“Yes, sounded good in here." confirmed Evans.
“Okay, Houston.” announced Cernan. "We’re hard docked.”
After the flight, Evans discussed how this docking differed from earlier in the mission when the LM was still attached to the S-IVB. “One of the noticeable differences between this docking and the docking with the S-IVB is the fact that the ascent stage did dance a lot more than the S-IVB did. The S-IVB is steady as a rock. The LM dead band would change attitude, and you’d try to follow it.”
In view of the difficulties that Apollo 14 had experienced w hen Stu Roosa tried to dock with the LM An tares while it was still on the S-IVB. his post-rendezvous docking was approached with some apprehension by mission control. Before the lunar landing. Bruce McCandless at the Capcom console gave the crew’ a change to the procedures. “With respect to docking, again we anticipate normal operation. However, we’d like to add to the normal procedures a LM plus-.v thrust of 10 seconds, four-jet RCS. to facilitate or to give us just a little more of a warm feeling on the docking.”
By having the LM thrust towards the CSM at the same Lime as Roosa was docking was evidently something neither Shepard nor Roosa was happy with. “We mutually agreed that it would be better to give it one go at least using the normal technique with no thrusting.” explained Shepard after the flight.
“We really didn’t see any advantage to that LM thrusting," added Roosa. "I didn’t like that idea of the LM coming on with thrust. We didn’t see where we had anything to lose by trying the normal docking method. If it didn’t capture, then we’d try it." In the event, Roosa’s docking went smoothly, without any hint of the troubles that had beset their earlier docking.