By the late 1960s and early 1970s, the Moon landings became the grand travel narratives of human history. Mars has subsequently emerged as the next great travel destination. There are multiple reasons why the planet so intrigues us. Through MER and other missions, Mars has become familiar and tangible. While our oceans obscure 70 percent of Earth’s topography, on Mars we can visually survey the entire surface, so that planetary scientists to some extent have a better understanding of its surface than of our home planet. Global Surveyor’s multi-colored elevation maps, for instance, reveal that Mars’s northern hemisphere lies low in elevation compared to the southern highlands. Far fewer impact craters in the northern hemisphere may indicate that at one time this region was covered by a primordial sea. Prominent on the Tharsis bulge is the tallest volcano in the Solar System, Olympus Mons (figure 3.5), accompanied by three nearby shield volcanoes. Looking toward Mars’s north pole, Arsia Mons is the most southern, then Pavonis Mons, and finally Ascraeus Mons. These adjacent volcanoes occur in a chain-l ike sequence reminiscent of the tectonic plate movement that produced the Hawaiian Islands, which raises the question of whether geothermal processes are still at work on Mars.
Moreover, extremophiles may thrive in possible underground water reserves, deep in the crust, or at the Martian polar caps. Re-
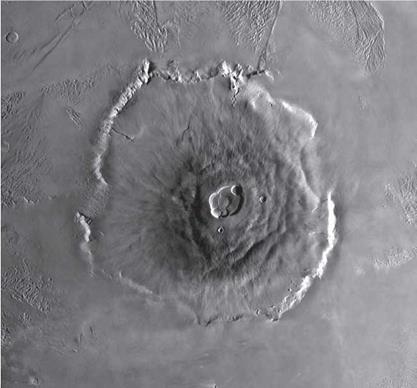
Figure 3.5. Olympus Mons rises to 69,480 feet above the Martian surface, is the largest volcano in the Solar System, and is a widely known and celebrated feature of the red planet. Nonetheless, Mars is so small that it only has mild tectonic activity and is almost dead, geologically speaking. Venus and Earth have much stronger volcanism. The width of the image is 1,000 km (NASA/Jet Propulsion Laboratory).
|
searchers have detected methane in the atmosphere, which could suggest active volcanism or a more exciting, possible microbial source.49 Despite Mars’s tenuous atmosphere, images by the Mars Reconnaissance Orbiter indicate groundwater sporadically breaks through the surface to briefly flow down steeply sloped terrain, and data from the 2008 Phoenix Lander indicate its soil is similar in acidity to Terran soil. Added to this are recent findings that ancient fossilized life on Earth, dating to more than 3.4 billion years ago, metabolized and lived on sulfur instead of oxygen, as Earth’s atmosphere was not then oxygen-rich.50 All of this suggests that Mars could harbor life deep within its ruddy dirt. If the Mars
Science Laboratory’s rover Curiosity finds evidence of ancient or extant extremophiles, we might discover how life emerged on the early Earth.
The other tremendous appeal of Mars is that it is the only planet astronauts could travel to, and explore, in the near future. Alluding to the passion with which we dream of stepping foot on worlds beyond our Moon, Ray Bradbury has observed, “We know not why we thread an architecture of travel in a fiery path across a winter space and warm far worlds with our breath.”51 Perhaps we do so simply because we survive on our own relatively cold planet only as a result of technology. Anthropologist Ben Finney points out that we inhabit the globe, from pole to pole, solely with the aid of our technological ingenuity. Finney contends that the Moon or Mars represent a logical next step for human migration. He researched the ocean routes Polynesian peoples navigated via canoe over as much as 1,000 miles of open ocean, from South China and Southeast Asia to Hawaii and other islands, to demonstrate how humans might hopscotch to nearby planets. Finney has shown that Polynesian peoples sailed with all the supplies they needed to establish themselves on islands that they could have only speculated existed but had never charted or seen. This was an enormous feat without even the aid of a simple compass or sea charts. And yet, they accomplished it using horizon markers and the rising and setting of the Sun and stars.
The Polynesians succeeded, asserts Finney, precisely because “[t]hey did not regard the ocean as an alien environment, but one which was utterly natural—and essential to the spread of human life.”52 Even as ancient seafarers ventured far beyond known shores to settle uninhabited islands in the wastes of the Pacific, Finney argues that we could and should strike out across the Solar System. The extremely challenging act of humans venturing to Mars or an asteroid could mark the first step. Noted physicist Stephen Hawking would agree. Hawking contends that the future of our species depends on our becoming a truly space-faring species as a means of surviving natural or human-produced global catastrophes.
Archaeologist Peter Capelotti observes that extreme exploration, “begun more than two million years ago with a few rough stone tools, in what would become Africa’s Olduvai Gorge, would culminate in Apollo 11.” Our desire for travel and exploration will take us far beyond Earth, suggests Capelotti:
By the time a human finally claimed to have stood at the North Pole, that icy coordinate had come to signify—and continues to represent—the human search for something larger in our culture and ourselves: the sense and the knowledge that our journey is a continuous one, and that the geographic “firsts” we credit to ourselves are merely waypoints on a continuum begun two million years ago. If our culture. . . (and, increasingly, our robots) allow it, this path may continue for millions of years more. We have set human feet upon only one North Pole; in space, there are millions of North Poles waiting to be discovered.53
As of this writing, Opportunity continues sampling the Martian terrain, and with each turn of its wheels, we take in more of the landscape, not just in images downloaded to NASA’s archives or as attached links to Google Mars, but into the human imagination and into our dreams. As Capelotti indicates, there are many mountaintop vistas in our Solar System yet to be glimpsed, through the stereoscopic eyes of our robotic co-explorers and, eventually, through our own eyes. The sublime heights of Martian volcanoes entice the explorer in us. Mountain climbers and space enthusiasts dream of summiting Olympus Mons, rising 69,480 feet above the Martian surface, and imagine the sweeping vistas they would take in from the rim of its calderas, or from the top of nearby Ascraeus Mons reaching 59,699 feet, or Arsia Mons’s summit of 57,085 feet.54 Our tallest peaks above sea level pale in comparison, with Mount Everest reaching a mere 29,035 feet. Rock climbers likewise anticipate the grandeur of venturing into Valles Marineris, extending more than 2,500 miles in length and whose canyon walls soar from three to six miles high. But we don’t have to wait for a brave explorer to rappel over the six-mile vertical scarp of Olympus Mons. Robotic eyes are even now taking us there.
The journey to, and across, Mars’s cracked and cratered terrain is one of the great travel narratives of the twenty-first century. With our rovers, we have alighted upon and are motoring through its fascinating desertscapes. In July 1997, the Pathfinder Mission successfully landed the Sojourner Rover at Ares Val – lis. Project scientist Matthew Golombek commented that, at the time, “an entire generation had never witnessed a landing on another planet” and reported that within the first month of operation, roughly 566 million people accessed the mission website.55 The twin MER rovers captivated even larger audiences. As science studies scholar Robert Markley points out, “Sojourner had traveled a few yards to take readings of the mineral composition of several rocks; the 2004 rovers provided a photographic record that seemingly mimicked the experience of humans walking across a Martian landscape reminiscent of terrestrial deserts.” Markley characterizes Spirit and Opportunity’s images as “the first vacation photographs from another world” that in turn have become “part of the semiotics of human visualization.”56 Through these rovers, we’ve sampled the planet’s sedimentary and volcanic rocks, as well as its ubiquitous dust. We’ve sniffed the air, measured barometric pressure, tasted the soil to determine its alkaline or saline qualities, and bored into rocks searching for fossilized evidence of water. On balmy afternoons, by Martian standards, we glimpsed the simultaneous dance of multiple dust devils. We’ve witnessed silent sunrises, seen morning fogs cling to the valleys and dissipate, and watched from Husband Hill through Spirit’s cameras as the Sun sank below the horizon. In winter, the rovers weathered the cold, dim days and we too waited them out. Rather than our rovers exploring with us, it seems that we’ve tagged along with them as they traverse, photograph, and engage with this captivating and stark landscape.
Humans have a presence on Mars, even if for now it is limited to remote-controlled rovers ambling over its arid plains. Little could Steve Squyres know that his sturdy MER rovers would continue doing science for years beyond their ninety-day mission. Squyres wonders about the astronauts who might someday find the derelict rovers: “Above all, I simply hope that someone sees them again,” writes Squyres. “Spirit and Opportunity have become more than just machines to me. The rovers are our surrogates, our robotic precursors” until astronauts someday leave their “boot prints in our wheel tracks at Eagle Crater.”57 Those astronauts are just as likely to find remnant tracks of Mars Science Laboratory’s rover Curiosity. If W. H. Auden was right in his poem “Moon Landing” about the eventuality, from the first flaked flint, of humans walking on the Moon, then boot prints on Mars seem equally probable— even inevitable.58