The Aurora Borealis and Aurora Australis occur when electromagnetic particles from the solar wind collide with or excite atoms in the Earth’s magnetosphere (figure 7.4). We now understand the
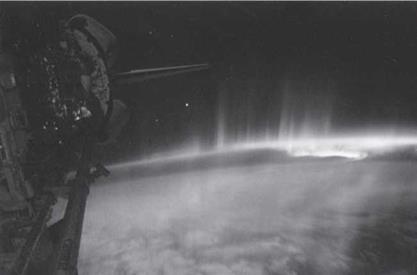
Figure 7.4. Aurora Australis as seen from the Space Shuttle, near the solar activity maximum that occurred in 1991. The aurora is caused by sheets of glowing gas, energized by high-energy solar radiation, and it extends up to an altitude of 300 miles. The light show is accompanied by more damaging effects to orbiting spacecraft and satellites (NASA/Earth Science and Image Analysis Laboratory).
|
explicit connection between auroras and magnetic storms, and recently have confirmed the “three-century old theory that auroras in the northern and southern hemispheres are nearly mirror images—conjugates—of each other.”38 Very few people in human history have inhabited the high (or low) latitudes where these brilliant light displays are usually visible. For thousands of years the ethereal, vertical curtains of light, draped from 60 to 200 miles above the Earth’s polar regions, have inspired wonder, as well as poetic and musical responses. The Vikings first recorded the Aurora Borealis in AD 1250. About seven hundred years later, in 1897, Norwegian explorer Fridtjof Nansen wrote of the northern lights: “It was an endless phantasmagoria of sparkling color, surpassing anything that one can dream. Sometimes the spectacle reached such a climax that one’s breath was taken away; one felt that now something extraordinary must happen—at the very least the sky must fall.”39
The twentieth-century composer Edgard Varese worked with “found sound,” incorporating sounds with a non-musical origin
into his music, and with electronic instruments such as the Theremin. He once composed a piece based on having seen the Aurora Borealis. His wife Louise recalled in her memoir of the composer, “Nature in its most magnificent and terribly impersonal aspects moved him passionately.” Titles for many of his compositions were drawn from astronomy or science. Of the aurora, Louise writes that Varese claimed he “not only saw but heard” the majestic luminescent curtains dancing across the night sky and later notated “the sounds that had accompanied the movements of the light.”40 Electromagnetic waves or natural radio waves can only be detected with a radio receiver.41 If we take Varese at his word, perhaps he experienced some form of synesthesia, so that in fact he had heard the northern lights. In her memoir, Louise Varese speculated that the score of the aurora her husband mentioned was either Les Cycles du Nord or Mehr Licht, two of several compositions that were later lost or destroyed.
More recently, Terry Riley and the Kronos Quartet’s chamber music composition Sun Rings, inspired by the Sun’s dynamic magnetic field, was written to be performed against a backdrop of IMAX-sized images from SOHO and the TRACE (Transition Region and Coronal Explorer) satellite. Riley’s score is accompanied by magnificent sunspots tracking across the face of the Sun as well as finely detailed footage of magnetic field lines breaking through, looping above, and re-submerging into the Sun’s surface. Such contemporary compositions speak to our deep, primal entanglement with the Sun, which is no less important to our lives now than it was in the ancient past.
From prehistory to the modern era, humans intuitively understood the Sun as integral to their very existence. Paleolithic and Neolithic communities scattered across the globe knew that the Sun powerfully shaped their lives. Evidence of their sense of the Sun’s significance is found at the Mnajdra temple on Malta, at Newgrange in Ireland, at Stonehenge on Salisbury Plain, and at many other megalithic structures. Such monuments speak of an ancient past in which peoples from disparate times and places were attentive and accurate observers of our star.
On the island of Malta, just off the coast of Sicily, are the remains of a complex of stone temples known as Mnajdra, dating to 5500 BC. One of these limestone edifices marks precise alignments with the Sun during fall and winter equinoxes.42 Physicist Guilio Magli describes Mnajdra as a “stone calendar” marking spring and autumn equinox: “In the course of the seasons, one can follow the movement of the Sun, which rises on the horizon, observing day by day at which point the light strikes the altar inside the temple.”43 In walking through Mnajdra, sited as it is overlooking the Mediterranean Sea, it’s easy to recognize that the location and the megalithic temple were sacred to its ancient architects. Built with cleverness but no metal tools, the effort involved in creating the edifice was prodigious, and a reminder of the investment ancient peoples made in giving homage to the Sun.
Stonehenge, the remarkable structure ancient Britons built on Salisbury Plain sometime between 3015 and 2400 BC, is so well aligned that “the general orientation of the axis of the monument [looks] . . . towards sunrise at the summer solstice in one direction, and towards sunset at the winter solstice in the other.”44 Caroline Alexander observes that, in coming upon Stonehenge, “the greatshouldered silhouette is so unmistakably prehistoric that the effect is momentarily of a time warp cracking onto a lost world.” Alexander contends that the architraves atop the monoliths, “bound to their uprights by mortise-and-tenon joints taken straight from carpentry, [are] an eloquent indication of just how radically new this hybrid monument must have been. It is this newness, this assured awareness that nothing like it had existed before, this revelatory quality that is still palpable in its ruined stones.” Though the stone temples at Gobekli Tepe in Turkey are far older and date to approximately 9600 BC, no prehistoric structure like Stonehenge exists anywhere else in the world. Alexander surmises that the Britons who constructed the monument “had discovered something hitherto unknown, hit upon some truth, turned a corner— there is no doubt that the purposefully placed stones are fraught with meaning.”45 Emphasizing the point that the architects deliberately aligned Stonehenge to mark the winter and summer solstices, Magli writes, “This is therefore the only information that the builders left us in writing. Granted it is written in stone, and with stone, and in the language of the sun and of the stones. But it is nevertheless written.”46
Diodorus of Sicily, a Greek historian from the first century BC, supposedly commented on “a lost account set down three centuries earlier, which described ‘a magnificent precinct sacred to Apollo and a notable spherical temple’ on a large island in the far north, opposite what is now France.”47 Stonehenge, Diodorus suggested, was constructed to pay homage to the Sun. As with the relics of any prehistoric culture, multiple interpretations are possible. Archaeologist Mike Parker Pearson of the Stonehenge Riverside Project has recently reinterpreted Stonehenge as a burial monument. He contends that the people who raised the monoliths on the chalk downs apparently gathered each winter solstice to mark the setting Sun as the beginning of a new year and to remember their ancestors.
Astronomer Edward Krupp has investigated the sophisticated and sizeable burial chamber in Ireland known as Newgrange or Bru na Boinne: “Newgrange is a megalithic surprise,” writes Krupp, who characterizes the structure as emerging from the landscape “like a highway tourist attraction.”48 During winter solstice at this stone monument, the first rays of the Sun illuminate a room deep in the structure to mark the beginning of the new year. Dating from about 3700 to 3200 BC, Newgrange was designed so that “two weeks either side of the winter solstice, the Sun, on rising, shown down the length of the entrance passage and illuminated the central chamber—as it still does.”49 The beam of sunlight, as it travels deep into the monument, is thinned and sculpted by the megaliths’ calculated placement. To offer a vivid picture of the massive effort required to construct the edifice, Magli emphasizes that “5000 years ago, someone built a monument involving thousands of tons of earth and rock, covered it with quartz like a giant jewelry box, [and] carefully measured the direction of the sunrise at winter solstice to line up a corridor built with stones as heavy as many elephants together.”50 Not surprisingly, the legend associated with Newgrange recounts tales of the earliest known Irish gods, “The Lords of Light.”51
The Chankillo complex in Peru predates the Inca by two thousand years, offering insight into the precursors to Sun worship among the Inca and their official Sun cult. In 2007, Ivan Ghe – zzi, Peru’s national director of archaeology, and astronomer Clive
Ruggles reported discerning the layout of what is considered the oldest solar temple in the Americas. Ghezzi was first to surmise the purpose of thirteen towers on a ridge near Chankillo, a fourth century BC ceremonial complex in the northern coastal, desert region of Peru. Traditionally considered a fortress, Chankillo is now understood to be a very precise solar observatory built by the people who predated the Inca. Ghezzi realized that the prominent line of stone towers were markers that indicated the Sun’s position throughout the months of the calendar year. He contacted Ruggles and together these researchers, using hand-held GPS devices, determined that the location of the towers as projected against the horizon “corresponds very closely to the range of movement of the rising and setting positions of the Sun over the year.” In particular, winter and summer solstice alignments are clearly marked by the towers. Ghezzi and Ruggles have shown that the towers and the gaps between them offered “a means to track the progress of the Sun up and down the horizon to within an accuracy of two or three days.”52
One import of the findings at Chankillo, write Ghezzi and Ruggles, is that “sunrise ceremonies, at a sanctuary on the Island of the Sun in Lake Titicaca, surrounding a crag regarded as the origin place of the Sun, almost certainly had pre-Incaic roots.”53 Either the Inca, or the peoples who predated them, named an island in Lake Titicaca as Isla del Sol, or Island of the Sun, in honor of the god who created the Sun, Moon, stars, and humankind. It is well known that at the high Andean city of Machu Picchu, constructed in the late 1400s, the Inca kept accurate records marking the winter solstice, the first day of the Inca year. But the Inca may have adopted their attentiveness to the Sun from peoples who far predated their great civilization.
Long before the Neolithic Britons were raising those remarkable trilithons on Salisbury Plain, the Egyptians had already developed writing and record keeping and were constructing the Great Sphinx at Giza, apparently in recognition of the god Horus or Horemakhet, believed to be a personification of the Sun on the horizon.54 An even earlier instantiation of the Sun god Horus was Ra, the patron god of the ancient Egyptian city Heliopolis, located in the Nile delta. In approximately 2400 BC, Ra was combined with the god of Thebes to become Amun-Ra, the highest deity in the Egyptian pantheon. These are a few of the varied civilizations that recognized the importance of the Sun to their survival. In the Information Age, we’re just as dependent on our star as ancient peoples—maybe even more so.