From time immemorial we have projected our stories, myths, and legends onto the night sky. Seeing patterns among the stars was a serious preoccupation from primordial time. Our shared narratives about the constellations, across cultures and millennia, served as a survival mechanism not to be underestimated or discounted as simple tales of mythological figures. Biocultural theorist Brian Boyd claims that human attention to pattern emerged as an evolutionary adaptation. Boyd and other scholars contend that arts relying on patterns such as storytelling, song, and cave painting emerged via natural selection and allowed individuals and clans to better collaborate and share information that enhanced survival.4
Recent analyses of prehistoric paintings and markings in caves in France have revealed a series of twenty-six symbols that may reflect humankind’s earliest attempts at pictographic writing, traditionally thought to begin about 3,000 BC. Anthropologists Genevieve von Petzinger and April Nowell collated a database of cave signs from 146 sites in France dating from 35,000 to 10,000 years ago. “What emerged was startling: 26 signs, all drawn in the same style, appeared again and again at numerous sites.”5 The symbols range from straight lines, to circles, spirals, ovals, dots, Xs, wavy lines, and various hand symbols among others. Similar symbols have been found at Paleolithic sites around the world.
Certain symbols studied in France frequently appear in deliberate groupings, such as the occurrence of dots with a particular hand symbol, which may indicate the beginnings of a system of writing. As Nowell speculates, “We are perhaps seeing the first glimpses of a rudimentary language system.”6
The celebrated Lascaux cave paintings not only incorporate these symbols, but may also include representations of the Pleiades and Hyades star clusters and an ice age panorama of the night sky. Clive Ruggles and Michel Cotte, who in 2010 headed the International Astronomical Union’s Working Group on Astronomy and World Heritage, reported to UNESCO that some archaeoas – tronomers contend a series of dots above the aurochs in the Hall of the Bulls represents the Pleiades star cluster and that one of the auroch’s eyes and adjacent dots may depict the star Aldebaran and the Hyades cluster.7 The French government’s website on Las – caux’s cave art notes that in all of the renderings, the horses were painted first, then the aurochs, and then the stags. These animals apparently correspond to the seasons of spring, summer, and autumn, respectively, providing “a metaphoric evocation that, in this setting, links biological and cosmic time.”8 Even more fascinating is Chantal Jeques-Wolkiewiez’s assertion that two panels of these cave images may depict the night sky as perceived by Magdalen – ian people from the top of Lascaux hill during a summer solstice roughly 17,000 years ago. Besides comparing the cave paintings to computer models of the night sky in the last ice age, she also found that light during sunset at the summer solstice stills enters the cave to illuminate some of the paintings.9
While it may be very difficult to determine whether the dots in the Lascaux paintings are indeed asterisms, the markings ice age peoples at Lascaux left next to their remarkable paintings enticingly suggest they were meaningful forms of communication. Extrapolating from such biocultural and archaeological research, it seems likely that our attention to patterns seen in constellations in the night sky extends back to our earliest days.
The names of stars and designation of constellations as we know them in Western culture are so ancient that their origins remain elusive.10 Long before the earliest written records, humankind told narratives about the stars and clustered them into constellations, which served as mnemonics for travel and navigation and as a repository of knowledge about the seasons, but also of legends and myth. Only in the last 3,600 years do we find unequivocal evidence of tracking the stars. Chinese rulers employed court astronomers to record information regarding stellar motions, transients, and astronomical events such as supernovae. But even older than ancient Chinese records is the Nebra sky disc. Discovered near the town of Nebra in Germany, the Nebra sky disc is believed by archaeo – astronomers to be a Bronze Age durable sky map dating back to 1600 BC.
The disc was uncovered in 1999 by treasure hunters who hit upon an ancient burial site in a circular earthwork enclosure at the top of Mettelberg Hill. The disc, 30 centimeters or 11.8 inches in diameter, was found with two bronze swords among other items. The sky disc is made of bronze with gold overlays of the Sun, the Moon in phase, and multiple stars. Gold bands on its sides indicate the east and west horizons and mark an angle of 82.5 degrees. At Nebra, sunset at the winter and summer solstices is visible on the horizon 82.5 degrees apart. As the angular separation of those setting points varies at differing latitudes, some ar – chaeoastronomers are convinced that the disc was constructed in the Nebra region and is the oldest extant sky map in the world. A cluster of seven gold dots on the disc are thought to be the earliest known representation of the Pleiades star cluster, used in the ancient past for identifying seasons of planting and harvest (figure 8.1). Investigation of its metal composition traces the disc to a Bronze Age mine in the Alps. The site where the disc was found is only 15 miles from Goseck, Germany, the location of a Neolithic ceremonial woodhenge dating to 7,000 years ago that researchers say clearly marks the position of sunrise on the horizon on the summer and winter solstices. Archaeologist Harald Meller, who posed as a buyer and worked with Swiss police in a sting operation to capture underground traders attempting to sell the sky disc, points out that it predates “the beginning of Greek astronomy by a thousand years.”11
The ancient Greek writers Homer and Hesiod knew the names of recognizable stars and star clusters like the Pleiades and Hyades, and of constellations such as Ursa Major, the Bear. The Iliad and The Odyssey, attributed to the poet known as Homer, remain the
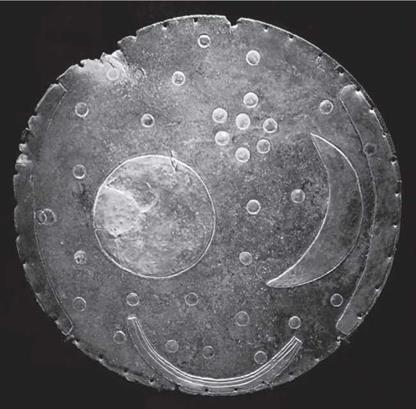
Figure 8.1. The Nebra Sky Disc dates to 1600 BC and is considered the oldest extant map of the Pleiades. Star-mapping was practiced by many ancient cultures, some of which left artifacts with realistic representations. The Pleiades is a nearby, young star cluster still embedded in the gauzy, glowing gas from which it formed, shown as a clump of points just above and to the right of the center of the disc (Wikimedia Commons/Dbachmann).
|
oldest extant Greek texts we have. Homer’s tales were rooted in an oral tradition that extends back centuries prior to their being recorded. In The Iliad, traditionally dated to approximately 700 BC, some of the constellations we know today appear on the shield that Hephaistos forged for Achilles:
He made the Earth upon it, and the sky, and the sea’s water, and the tireless Sun, and the Moon waxing into her fullness, and on it all the constellations that festoon the heavens,
The Pleiades and the Hyades and the strength of Orion and the Bear.12
James Evans also notes Homer’s description of Odysseus orienting his ships by keeping the constellation Ursa Major, which turns about the celestial north pole, left of his vessels as he sails East.13
One significant reason early agrarian societies marked the stars in the night sky was to develop an agricultural calendar, initially important for planting and harvesting. Evans points out that a few generations after Homer, Hesiod wrote Works and Days, the opening lines of which directly associate the rising and setting of star groups with agriculture:
When the Pleiades, daughters of Atlas are rising,
Begin the harvest, the plowing when they set.14
Evans explains that winter wheat was the only wheat planted in Greek antiquity, so that when the Pleiades were setting in the west in late fall it was time to plow the ground and plant the wheat.
Nick Kanas points out that ancient Greek astronomers supplemented their knowledge of the night sky with what they could glean from the Egyptians and Babylonians: “From the Egyptians, they learned about the length of the year, its break-up into a 12-month calendar, the division of day and night into 12 hours each. . . . From the Mesopotamians, they learned a sophisticated system of constellations[,] especially involving the zodiac along the ecliptic.”15 Babylonian temple scribes conducted serious astronomical observation and carefully preserved their records on cuneiform tablets. Many of these astronomical records have been recovered, some of which date to the seventh century BC. A few of the most well known of these tablets are titled MUL. APIN, meaning “Plow Star,” the title of which apparently refers to the stars of the Triangulum constellation and the star Gamma Androme – dae, not Ursa Major, also known as the Plough, Big Dipper, or the Bear. Evans observes that the MUL. APIN tablets, copies of much older texts, begin with a list of dozens of stars and provide a star calendar indicating the rising and setting of stars at particular times of the year. Also included in the tablets, which are more accurate than Hesiod’s agricultural calendar, are observations of various constellations as well as the planets Mercury, Venus, Mars, Saturn and Jupiter, the planet associated with the primary god of Babylon.
Ancient Greek astronomers thought of the stars as fixed or unmovable. However, the Greek astronomer Hipparchus, after whom ESA’s Hipparcos mission is named, was an accurate observer who “suspected that one of the stars may have moved, and. . . wished to bequeath to his successors data against which any future suspected movements might be tested.”16 Hipparchus was interested in what today is called astrometry, or the science of measuring the position and motions of stars and other astronomical objects. He produced a star catalog now lost or destroyed. According to Floor van Leeuwen: “The oldest catalog of stellar positions we know of is the compilation made around 129 BC by Hipparchus, a catalog that is still being investigated. Its only surviving copy appears to be a map of the sky on a late Roman statue, and is known as the Farnese Atlas.”17
For thousands of years, all we’ve known of Hipparchus’s star guide were descriptions by Ptolemy. But astronomer Bradley Schaefer asserts that, indeed, the Farnese Atlas (figure 8.2), a statue of the Greek figure Atlas kneeling while holding on his shoulders a globe of constellations, represents the stars and constellations known to the ancient Greeks. He contends that the statue “is the oldest surviving depiction of the set of the original Western constellations, and as such can be a valuable resource for studying their early development.”18 Schaefer realized after a detailed study of the globe that the constellations depicted match the night sky in the era and from the location where Hipparchus lived in 129 BC. As evidence in favor of this possibility, Schaefer writes: “First, the constellation symbols and relations are identical with those of Hipparchus and are greatly different from all other known ancient sources. Second, the date of the original observations is 125 ± 55 BC, a range that includes the date of Hipparchus’s star catalogue (c. 129 BC) but excludes the dates of other known plausible sources.” Schaefer concludes that “the ultimate source of the position information [of the constellations on the globe] used by the original Greek sculptor was Hipparchus’s data.”19
Hipparchus was the first to identify the Earth’s precession, produced by the gravity of the Sun and Moon on the Earth’s equatorial bulge. The “precession of the equinoxes” refers to the gravitationally induced, gradual shift in the Earth’s axis of rotation, so
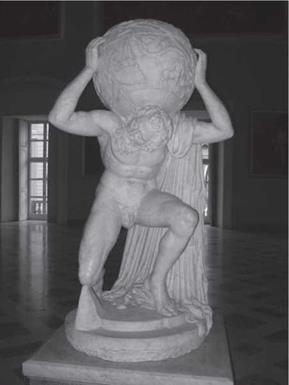
Figure 8.2. The Farnese Atlas is a marble statue from Roman times made from a Greek original, standing seven feet tall. Bradley Schaefer has argued that the star catalog of Hipparcus served as inspiration for the detailed constellations represented on the globe. This interpretation, and dates attached to the statue, remain controversial (Wikimedia Commons/Gabriel Seah).
|
that the equinoxes occur earlier each sidereal year over the course of 25,765 years, when this cycle of precession begins again. Precession is a changing view of the stars caused by a subtle variation in the Earth’s orbital orientation relative to the Sun; it’s not related to the kind of stellar movement that ESA’s Hipparcos mission has charted.20 As Michael Perryman explains, the Hipparcos mission is based on the concept of parallax: “The key to measuring stellar distances is actually based on the classical surveying technique of triangulation. It simply makes use of the fact, known since the time of Copernicus, that the Earth moves around the Sun, taking one year to complete its orbit. This yearly motion provides slightly different views of space as we speed around the Sun.” We experience the same effect in observing an object by first closing one eye and then the other. Perryman points out that “this stereo vision gives us depth perception and allows us to estimate distances, at least to nearby objects. . . . Astronomers use the same stereo technique, but with views of the celestial sky separated by hundreds of millions of kilometers as the Earth moves around the Sun. In this way, Nature has generously and serendipitously granted us the possibility of measuring distances stretching across the vast expanse of our Galaxy.”21