Before the defense establishment transferred the technology from Project Suntan to rocketry, it had to be nudged by a proposal from Convair’s Krafft Ehricke. Called to service in a Panzer division on the western and then eastern fronts during World War II, the young German was still able to earn a degree in aeronautical engineering at the Berlin Technical Institute. He was fortunate enough to be assigned to Peenemunde in June 1942, where he worked closely with Thiel. Although he came to the United States as part of von Braun’s group and moved with it to Huntsville, Ehricke was a much less conservative engineer than von Braun. Whether for that reason or others, he transferred to Bell Aircraft in 1952 when it was working on the Agena upper stage and other projects. Not happy there either by the time he left (when he believed interest had shifted away from space-related projects), he heeded a call from Karel Bossart to work at Convair in 1954.5
At the San Diego firm, Ehricke initially served as a design specialist on Atlas and was involved with Project Score. By 1956, he was beginning to study possible boosters for orbiting satellites but could find no support for such efforts until after Sputnik I. Then, General Dynamics managers asked him to design an upper stage for Atlas. (Consolidated Vultee Aircraft Corporation merged into General Dynamics Corporation on April 29, 1954, to become the Convair Division of the larger firm.) Ehricke and other engineers, including Bossart, decided that liquid hydrogen and liquid oxygen were the propellants needed. Ehricke worked with Rock – etdyne to develop a proposal titled “A Satellite and Space Development Plan." This featured a four-engine stage with pressure feeding of the propellants, neither Rocketdyne nor Ehricke being aware of Pratt & Whitney’s pumps. In December 1957, James Dempsey, vice president of the Convair Division, sent Ehricke and another engineer off to Washington, D. C., to pitch the design to the air force.6
The air service did not act on the proposal, but on February 7,
1958, Ehricke presented it to the new Advanced Research Projects Agency, created by the Department of Defense. For a time, ARPA exercised control over all military and civilian space projects before relinquishing the civilian responsibility to NASA in October 1958. Thereafter, for a year, ARPA remained responsible for all military space projects, including budgets. The new agency made Ehricke aware of Pratt & Whitney’s hydrogen pumps and encouraged Con – vair to submit a proposal using two 15,000-pound-thrust, pump-fed engines, which it did in August 1958. That same month, ARPA issued order number 19-59 for a high-energy, liquid-propellant upper stage to be developed by Convair-Astronautics Division of General Dynamics Corporation, with liquid-oxygen/liquid-hydrogen engines to be developed by Pratt & Whitney.7
In October and November 1958, at ARPA’s direction the air force followed up with contracts to Pratt & Whitney and Convair for the development of Centaur, but NASA’s first administrator, Keith Glen – nan, requested that the project transfer to his agency. Deputy Secre – 176 tary of Defense Donald Quarles agreed to this arrangement in prin – Chapter 5 ciple, but ARPA and the air force resisted the transfer until June 10,
1959, when NASA associate administrator Richard E. Horner proposed that the air force establish a Centaur project director, locate him at the Ballistic Missile Division in California, but have him report to a Centaur project manager at NASA Headquarters. NASA would furnish technical assistance, with the air force providing administrative services. The DoD agreed, and the project transferred to NASA on July 1, 1959. Lieutenant Colonel Seaberg from the Suntan project became the Air Research and Development Command project manager for Centaur in November 1958, located initially at command headquarters on the East Coast. Seaberg remained in that position with the transfer to NASA but moved his location to BMD. Milton Rosen became the NASA project manager. In November 1958, Ehricke became Convair’s project director for Centaur.8
Complicating Centaur’s development, in the fall of 1958 NASA engineers had conceived of using the first-stage engine of Vanguard as an upper stage for Atlas, known as Vega. NASA intended that it serve as an interim vehicle until Atlas-Centaur was developed. Under protest from Dempsey that Convair already had its hands full with Atlas and Centaur, on March 18, 1959, NASA contracted with General Dynamics to develop Atlas-Vega. With the first flight of the interim vehicle set for August 1960, Vega at first became a higher priority for NASA than Centaur. As such, it constituted an impediment to Centaur development until NASA canceled Vega on
December 11, 1959, in favor of the DoD-sponsored Agena B, which had a development schedule and payload capability similar to Vega’s but a different manufacturer (Bell).9
Besides Vega’s competition for resources until this point, another hindrance to development of Centaur came from liquid hydrogen’s physical characteristics. Its very low density, extremely cold boiling point, low surface tension, and wide range of flammability made it extremely difficult to work with. Ehricke had some knowledge of this from working with Thiel, but the circumstances of the contract with the air force limited the amount of testing he could perform to overcome hydrogen’s peculiarities.10
One limitation was funding. When ARPA accepted the initial proposal and assigned the air force to handle its direction, the stipulations were that there be no more than $36 million charged by Convair-Astronautics for its work, that a first launch attempt occur by January 1961, and that the project not interfere with Atlas development. At the same time, Convair was to use off-the-shelf equipment as well as Atlas tooling and technology as much as possible. Funding for the Pratt & Whitney contract was $23 million, bringing the total initial funding to $59 million for the first six launches the contract required, not including the costs of a guidance/control system, Atlas boosters, and a launch complex. Ehricke believed that, until it was too late, the limited funding restricted the necessary ground testing his project engineers could do. Also restrictive was the absence of the DoD’s highest priority (known as DX), which meant that subcontractors who were also working on projects with the DX priority could not give the same level of service to Centaur as they provided to higher-priority projects.11
Under these circumstances, Convair and Pratt & Whitney proceeded with designs for the Centaur structure and engines. The Centaur stage used the steel-balloon structure of Atlas, with the same 10-foot diameter. The lightness of the resulting airframe seemed necessary for Centaur because of liquid hydrogen’s low density, which made the hydrogen tank much larger than the oxygen tank. Conventional designs with longerons and ring frames would have created a less satisfactory mass fraction than did the pressurized tanks with thin skins (initially only 0.01 inch thick). The elliptical liquid oxygen tank was on the bottom of the stage. To create the shortest possible length and the lowest weight, the engineers on Ehricke’s project team made the bottom of the liquid-hydrogen tank concave so that it fit over the convex top of the oxygen tank.
This arrangement solved space and weight problems (saving about 4 feet of length and roughly 1,000 pounds of weight) but created oth-
ers in the process. One resulted from the smallness of the hydrogen molecules and their extreme coldness. The skin of the oxygen tank had a temperature of about — 299°F, which was so much “warmer" than the liquid hydrogen at — 423°F that the hydrogen would gasify from the relative heat and boil off. To prevent that, the engineers devised a bulkhead between the two tanks that contained a fiberglass – covered Styrofoam material about 0.2 inch thick in a cavity between two walls. Technicians evacuated the air from the pores in the Styrofoam and refilled the spaces with gaseous nitrogen. They then welded the opening. When they filled the upper tank with liquid hydrogen, the upper surface of the bulkhead became so cold that it froze the nitrogen in the cavity, thus creating a vacuum as the nitrogen contracted into the denser solid state, a process called cryopumping.12
Because of the limited testing, it was not until the summer and early fall of 1961 that the Centaur engineers and managers learned of heat transfer across the bulkhead that was more than 50 times the amount expected. It turned out that there were very small cracks in the bulkhead through which the hydrogen was leaking and destroy – 178 ing the vacuum, causing the heat transfer and resultant boil-off of Chapter 5 the fuel. This necessitated venting to avoid excessive pressure and explosion in the hydrogen tank. But the venting depleted the fuel, leaving an insufficient quantity for the second engine burn required of Centaur for coasting in orbit and then propelling a satellite into a higher orbit.
General Dynamics had used Atlas manufacturing techniques for the materials on the bulkhead. Atlas’s quality-control procedures permitted detection of leaks in bulkheads down to about 1/10,000 inch. Inspections revealed no such leaks, but the engineers learned in the 1961 testing that hydrogen could escape through even finer openings. Very small cracks that would not be a problem in a liquid – oxygen tank caused major leakage in a liquid-hydrogen tank.13
By the time Convair-Astronautics had discovered this problem, NASA had assigned responsibility for the Centaur project to the Marshall Space Flight Center (on July 1, 1960), with Seaberg’s Centaur Project Office remaining at BMD in California. Hans Hueter became director of Marshall’s Light and Medium Vehicles Office in July, with responsibility for managing the Centaur and Agena upper stages. During the winter of 1959-60, NASA also established a Centaur technical team following the cancellation of the Vega project. This team consisted of experts at various NASA locations to recommend ways the upper stage could be improved. In January 1960, navy commander W. Schubert became the Centaur project chief at NASA Headquarters.14
From December 11 to 14, 1961, John L. Sloop visited General Dynamics/ Astronautics (GD/A) to look into Centaur problems, particularly the one with heat transfer across the bulkhead. Sloop had been head of Lewis Laboratory’s rocket research program from 1949 until 1960, when he moved to NASA Headquarters. There in 1961 he became deputy director of the group managing NASA’s small and medium-sized launch vehicles. Following his visit, he wrote, “GD/A has studied the problem and concluded that it is not practical to build bulkheads where such a vacuum [as the one Ehricke’s team had designed] could be maintained." The firm also believed “that the only safe way to meet all Centaur missions is to drop the integral tank design and go to separate fuel and oxidizer tanks." Sloop disagreed: “If a decision must be made now, I recommend we stick to the integral tank design, make insulation improvements, and lengthen the tanks to increase propellant capacity."15
Sloop’s optimism was justified. After the Centaur team began “a program of designing and testing a number of alternate designs," tests revealed that adding nickel to the welding of the double bulkhead (and elsewhere), significantly increased the single-spot shear strength of the metal at —423°F.16
Centaur development experienced many other problems. Several of them involved the engines. After enduring “inadequate facilities, slick unpaved roads, mosquitoes, alligators, and 66 inches of rain in a single season" while developing the 304 engine for Suntan at West Palm Beach, Pratt & Whitney engineers also “discovered the slippery nature of hydrogen." The extreme cold of liquid hydrogen precluded using rubber gaskets to seal pipe joints, designers having to resort to aluminum coated with Teflon and then forced into flanges that mated with them. There had to be new techniques for seals on rotating surfaces, where carbon impregnated with silver found wide use. Another concern with the cryogenic hydrogen was that the liquid not turn to gas before reaching the turbopumps. The engineers initially solved that problem by flowing propellants to the pumps before engine start, precooling the system.17
The turbopump for the 304 engine used oil to lubricate its bearings. This had to be heated to keep it from freezing in proximity to the cold pump, creating a temperature gradient. To solve this problem for the RL10, the Pratt & Whitney engineers coated the cages holding the bearings with fluorocarbons similar to Teflon and arranged to keep the bearings cold with minute amounts of liquid hydrogen. This produced the same effect as lubrication, because it turned out that the main function of oil was to keep the bearings from overheating. The substance from which Pratt & Whitney nor-
mally made its gears, called Waspalloy, bonded in the hydrogen environment. Engineers replaced it with carbonized steel coated with molybdenum disulfide for dry lubrication. This solved the bonding problem but subjected some unlucky engineers to observing tests of the new arrangement by using binoculars from an observation post with only a screen door. Late at night, alligator croakings and other noises created uneasiness for many young observers unused to swamp sounds.18
The first component tests of the combustion chamber for the RL10, including stainless-steel regenerative-cooling tubes brazed with silver, took place in May 1959. As with many other initial tests of combustion chambers, there were signs of burnthrough, so the engineers changed the angle at which the hydrogen entered the tubes and aligned the tubes more carefully so they did not protrude into the exhaust stream. Engine firings two months after this showed that the changes had solved the burnthrough problem, but the chamber’s conical shape produced inefficient burning. Engineers changed the design to a bell shape and conducted a successful 180 engine run in September 1959, less than a year from the date of the Chapter 5 initial contract.19
A major innovation in the design of the RL10 took advantage of the cold temperature of liquid hydrogen in order to dispense with a gas generator to drive the turbopump. The cryogenic fuel passed from the tank into the tubes of the combustion chamber for cooling. As it did so, it absorbed heat, which caused the fuel to vaporize and expand. This provided enough kinetic energy to drive the turbine that operated both the liquid-hydrogen and liquid-oxygen pumps. It also provided the power for the hydraulic actuators that gimballed the engine. This process, called the “bootstrap" cycle, still used hydrogen-peroxide boost pumps to start the process. Hydrogen peroxide also powered attitude-control rockets and ullage-control jets that propelled the Centaur forward in a parking orbit and thereby forced the liquid hydrogen to the rear of the tanks. There it could be pumped into the engines for ignition.20
Before the RL10 underwent its first test in an upright position on a test stand in its two-engine configuration for the Centaur, it underwent 230 successful horizontal firings. It produced 15,000 pounds of thrust and achieved a specific impulse of about 420 lbf-sec/lbm at an expansion ratio of 40:1 through its exhaust nozzle. As required by its missions in space, it reliably started, stopped, and restarted so that it could coast in a parking orbit until it reached the optimum point for injection into an intended orbit (or trajectory for interplanetary voyages). On November 6, 1960, two RL10s, upright for the
first time on a test stand at the Pratt & Whitney facility in Florida, fired at the same time and did so successfully—for a short time until a problem occurred with the timer on the test stand. When engineers repeated the test the next day, only one engine fired. The other filled with hydrogen and oxygen until the flame from the first engine caused an explosion that damaged the entire propulsion system beyond repair.
A tape recording of the countdown suggested that the problem had stemmed from the faulty operation of a test-stand sequencer, so engineers did not suspect difficulties with the engine itself. By January 12, 1961, they repaired the test stand and tested another pair of engines. This time, they put a blast wall between the two engines and installed a shutoff valve on the hydrogen tank. They also separated the exhaust systems for the two engines by a greater distance. During this test, there was no problem with the sequencing, but the explosion recurred. In the vertical position, engineers learned, gravity was affecting the mixing of the oxygen with the hydrogen differently than it had in the horizontal position. So in a further instance of cut-and-try engineering, designers had to adjust the method of hydrogen feed. They also designed a method of measuring the density of the mixture to ensure the presence of enough oxygen for ignition. With these adjustments, the two engines fired simultaneously in the vertical test stand on April 24, 1961. Following this success, the engines completed 27 successful dual firings at Pratt & Whitney and 5 more at the rocket site on Edwards AFB in California. They then passed the flight-rating test from October 30 to November 4, 1961, in which they completed 20 firings equivalent in duration to six Centaur missions.21
To protect the liquid hydrogen in its tank from boiling off while the vehicle was on the launching pad and during ascent through the atmosphere, engineers had designed four jettisonable insulation panels made of foam-filled fiberglass. These were about a centimeter (0.39 inch) thick, held on the tank by circumferential straps. To keep air from freezing between the tank and the insulating foam, thereby bonding the panels to the tank, engineers designed a helium system to purge the air. To limit the weight penalty imposed by the panels (1,350 pounds), they had to be jettisoned as soon after launch as the atmosphere thinned and the ambient temperature dropped.22
Because of delays resulting from the engine ignition problem, difficulties with elaborate test instrumentation (such as a television camera and sensors inside the liquid-hydrogen tank), and other issues, an Atlas LV-3 with a Centaur upper stage did not launch for the first time until May 8, 1962, 15 months later than planned. The
THRUST- 15,000 LB (ALTITUDE) THRUST DURATION-470SEC SPECIFIC IMPULSE-433SEC ENGINE WT DRY-298 LB EXIT-TO THROAT AREA RATIO – 40 ТОЇ
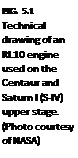
PROPELLANTS-LOX & LH2 PROPELLANT FLOW RATE – 35 LB/SEC
CONTRACTOR-
PRATT & WHITNEY SYSTEM-SAT I/S-1V (6 ENGINES) CENTAUR (2 ENGINES)
l-RM-D IND 8І4ЮВ
goals of the test flight were to proceed through the boost phase with jettison of the insulation and a nose fairing, followed by Centaur’s separation from the Atlas. With only a partial load of fuel, the Centaur was to coast for 8 minutes, reignite, and burn for 25 seconds.23
On the launch, the two stages rose normally until they approached maximum dynamic pressure (with resultant aerodynamic buffeting) as the vehicle got close to the speed of sound 54.7 seconds into the launch. Then, an explosion occurred as the liquid – hydrogen tank split open. Initially, engineers decided that aerodynamic forces had destroyed the insulation and ruptured the tank. About five years later, tests suggested that the real culprit was differential thermal expansion between a fiberglass nose fairing and the steel tank, causing a forward ring to peel off the tank.24
Even before this launch, the difficulties with engine development, resultant schedule delays, and problems such as the one with the bulkhead between the hydrogen and oxygen tanks had led to close scrutiny of the Centaur project and danger of its cancellation. Following John Sloop’s visit to General Dynamics to look into such problems, he had expressed concerns about the firm’s organization. Krafft Ehricke, the program director, had only five men reporting directly to him, and Deane Davis, the project engineer, had direct charge of only two people. Many other people worked on Centaur (27 of them full-time), but most of them were assigned to six oper-
ating divisions not directly under project control. Sloop wrote, “As far as I could tell in three days of discussion, the only people who have direct and up-to-date knowledge of all Centaur systems are Mr. Ehricke and Mr. Davis." Marshall Space Flight Center had “a very competent team of four men stationed at GD/A," and they were well aware of the “management deficiencies" emphasized in Sloop’s comments.25
Hans Hueter wrote on January 4, 1962, to GD/A president James Dempsey stating his concern about the way the Centaur Program Office was organized in “relation to the line divisions." He mentioned that the two of them had discussed this issue “several times" and reiterated his and other NASA employees’ “impression that the systems engineering is carried on singlehandedly by your excellent associates, Krafft Ehricke and Dean [sic] Davis." He added, “The individual fields such as propulsion, thermal and liquid behavior, guidance and control, and structures are covered in depth in the various engineering departments but coordination is sorely lacking."26
In response to NASA’s concerns about this matrix organization, Dempsey shifted to a “projectized" arrangement in which roughly 1,100 employees at Astronautics were placed under the direct authority of the Centaur program director. Ehricke was reassigned as the director of advanced systems and Grant L. Hansen became Centaur program director and Astronautics vice president on February 1, 1962. Trained as an electrical engineer at Illinois Institute of Technology, Hansen had worked for Douglas Aircraft from 1948 to 1960 on missile and space systems, including the Thor, with experience in analysis, research and development, design, and testing. He came to GD/A in 1960 to direct the work of more than 2,000 people on Atlas and Centaur. After February 1962, Ehricke continued to offer Hansen advice. Although he was imaginative and creative, the company had decided Ehricke “wasn’t enough of a[n] S. O.B. to manage a program like this." Hansen proved to be effective, although it is only fair to note that he was given authority and an organization Ehricke had lacked.27 S. O.B. or not, had Ehricke started with Hansen’s organization and adequate funding, Centaur development could have been smoother from the beginning. In any event, this sequence of events showed how management arrangements and technical problems interacted.
Several other programmatic changes occurred around this time. On January 1, 1962, for example, NASA (in agreement with the DoD) transferred the Centaur Project Office from Los Angeles to Huntsville, Alabama, and converted existing air force contracts to
NASA covenants. Lieutenant Colonel Seaberg ceased being project manager, and Francis Evans at Marshall Space Flight Center assumed those duties under Hueter’s direction. By this time, funding had grown from the original $59 million to $269 million, and the number of Centaur vehicles to be delivered had risen from 6 to 10.28
Meanwhile, following the May 8, 1962, explosion, a congressional Subcommittee on Space Sciences, chaired by Rep. Joseph E. Karth (D-Minnesota), began hearings on the mishap. In a report issued on July 2, 1962, the parent Committee on Science and Astronautics in the U. S. House of Representatives stated that “management of the Centaur development program has been weak and ineffective both at NASA headquarters and in the field."29 NASA did not immediately make further changes, but Marshall management of Centaur posed problems. These came out in the hearings, prompting unfavorable comment in the committee report. Von Braun had remarked about GD/A’s “somewhat bold approach. In order to save a few pounds, they have elected to use some rather, shall we say, marginal solutions where you are bound to buy a few headaches before you get 184 it over with." Hansen agreed that his firm was inclined “to take a Chapter 5 little bit more of a design gamble to achieve a significant improvement, whereas I think they [Marshall engineers] build somewhat more conservatively." The congressional report noted, “Such a difference in design philosophy can have serious consequences."30
Ehricke characterized the design approach of the von Braun team as “Brooklyn Bridge" construction. The contrast between that and the approach of General Dynamics appears in an account of a Marshall visit to GD/A that Deane Davis wrote at an unspecified date soon after Marshall took over responsibility for Centaur in July 1960. A group led by von Braun and including Hueter and structures chief William Mrazek had come to GD/A for a tour and briefings on Atlas and Centaur. Mrazek and Bossart had gotten into a discussion of the structure of the steel-balloon tanks, with Mrazek (according to Davis’s account) unwilling to admit that they could have any structural strength without ribs. Bossart took him out to a tank and handed him a fiberglass mallet containing lead to give it a weight of 7 pounds. It had a rubber cover and a 2-foot handle. Bossart invited Mrazek to hit the tank with it. After a tap and then a harder whack, he could not find a dent. Bossart urged him to “stop fiddling around. Hit the damned thing!" When Mrazek gave it a “smart crack," the mallet bounced back so hard it flew about 15 feet, knocking off the German’s glasses on the way and leaving only a black smear (no dent) on the tank. Davis wrote that Hueter was as amazed as Mrazek by the strength of the tank.31
This account is difficult to accept entirely at face value because Mrazek had already designed the Redstone with an integral-tank structure that was hardly as light as Bossart’s steel balloon but was also not quite bridgelike. Nevertheless, even in 1962 von Braun was clearly uncomfortable with Bossart’s “pressure-stabilized tanks," which he called “a great weightsaver, but. . . also a continuous pain in the neck" that “other contractors, for example the Martin Co., for this very reason have elected not to use." No doubt because of such concerns, von Braun sought quietly to have the Centaur canceled in favor of a Saturn-Agena combination.32
Faced with this situation, on October 8, 1962, NASA Headquarters transferred management of the Centaur program to the Lewis Research Center, to which Silverstein had returned as center director in 1961 from his position at NASA Headquarters. A “sharp, aggressive, imaginative, and decisive leader," Silverstein could be “charming or abrasive," in the words of John Sloop. Deane Davis, who worked with him on Centaur, called him a “giant among giants" and a man he “admired, adored, hated, wondered about—and mostly always agreed with even when I fought him. Which was often." Under Silverstein’s direction, the Lewis center required much more testing than even the Marshall group had done. Lewis tested everything that could “possibly be proven by ground test." Yet despite such aggressive oversight, Grant Hansen expressed admiration for Lewis and its relationship with his own engineers.33
Because the RL10 had been planned for use on Saturn as well as Centaur, its management remained at Marshall. The reason given for Centaur’s transfer was that it would allow the Huntsville engineers to concentrate on the Saturn program. A NASA news release quoted NASA administrator James Webb, “This, I feel, is necessary to achieve our objectives in the time frame that we have planned. It will permit the Lewis Center to use its experience in liquid hydrogen to further the work already done on one of the most promising high energy rocket fuels and its application to Centaur. . . ."34
Long before this transfer, engineers from the Cleveland facility had been actively involved in helping solve both engine and structural problems with the vehicle. Their involvement included use of an altitude chamber at their center. Other facilities, including a rocket sled track at Holloman AFB, New Mexico, had also been involved in Centaur development. For example, in 1959 GD/A had done some zero-G testing in an air force C-131D aircraft at Wright- Patterson AFB (and also, at some point, in a KC-135). The same year, the firm had acquired a vacuum chamber for testing gas expansion and components. With additional funding (to a total of
about $63 million) in 1960, GD/A extended testing to include use of the vacuum test facility at the air force’s Arnold Engineering Development Center in Tullahoma, Tennessee, zero-G test flights using Aerobee rockets, and additional static ground testing, including modifying test stand 1-1 at the rocket site on Edwards AFB for Centaur’s static tests. In 1961, when GD/A’s funding rose to $100 million, there were wind-tunnel tests of the Centaur’s insulation panels at NASA’s Langley Research Center, additional zero-G testing, and construction of a coast-phase test stand to evaluate the attitude-control system.35
At Lewis, Silverstein decided to direct the Centaur project himself, assisted by two managers under his personal direction and some 41 people involved with technical direction. Some 40 Marshall engineers helped briefly with the program’s transition. By January 1963, the changeover was mostly complete and Centaur had acquired a DX priority. Then, costs for Centaur were estimated at $350 million, and containing them became an issue. Despite this, Silverstein decided that the first eight Centaurs after the transfer 186 would constitute test vehicles. By this time, Surveyor spacecraft Chapter 5 had been assigned as Centaur payloads, and Silverstein determined that none of them would be launched until the test vehicles had demonstrated Centaur’s reliability.36
By February 1963, Silverstein had appointed David Gabriel as Centaur manager but placed the project office in the basement of his own administrative building so he could continue to keep tabs on the project. Some continuity with the period of Marshall management came in the retention of Ronald Rovenger as chief of the NASA field office at GD/A. Instead of 4, his office rose to a complement of 40 NASA engineers. It took until April 1964, but Lewis renegotiated the existing contracts with GD/A into a single cost- plus-fixed-fee document for 14 Centaur upper stages plus 21 test articles. The estimated cost of the agreement was roughly $321 million plus a fixed fee of $31 million, very close to the estimate of $350 million at the beginning of 1963. However, Silverstein felt the need for a second contract to cover further modifications resulting from Lewis’s technical direction. Soon the Lewis staff working on Centaur grew to 150 people. Silverstein continued to give the project his personal attention and made a major decision to abandon temporarily the use of a parking orbit and restart for Surveyor. This required a direct ascent to the Moon, considerably narrowing the “window" for each launch.37
These and other changes under Lewis direction did not immediately solve all of Centaur’s problems. Test flights and resultant
Date
|
Mission
|
Objective
|
Outcome
|
Nov. 27,
|
R&D,
|
Achieve separation
|
Successful,
|
1963
|
single-burn
|
of Centaur, Earth
|
achieved
|
|
|
orbit, data on nose
|
orbit close to
|
|
|
cone, insulation
|
that planned,
|
|
|
panels
|
gathered data
|
June 30,
|
R&D,
|
Test jettison
|
Jettison
|
1964
|
single-burn,
|
of redesigned
|
successful
|
|
restart
|
insulation panels
|
but failure of
|
|
boost
|
and nose cone,
|
driveshaft in
|
|
pumps
|
gather data from
|
hydraulic pump
|
|
|
restart
|
prevented
gimballing
|
Dec. 11,
|
R&D,
|
Restart engines,
|
Partial success;
|
1964
|
two-burn
|
carry Surveyor
|
first burn
|
|
|
model
|
successful but ullage motors not powerful enough to keep LH2 at bottom of tank;a weak
|
|
|
|
restart
|
Mar. 2,
|
R&D,
|
Simulate Surveyor
|
Failed; Atlas fuel
|
1965
|
single-burn,
|
launch
|
valve closed,
|
|
separable
|
|
causing an
|
|
Surveyor
model
|
|
explosion
|
Aug. 11,
|
R&D,
|
Demonstrate
|
Successful in
|
1965
|
single-burn,
|
capability of
|
separating
|
|
separable
|
launching Surveyor
|
model and
|
|
Surveyor
|
model similar to
|
sending on
|
|
model
|
actual spacecraft
|
planned course
|
Apr. 7,
|
R&D,
|
Perform 25-minute
|
Partial failure;
|
1966
|
two-burn,
|
coast in parking
|
in parking
|
|
separable
|
orbit, re-ignite
|
orbit there was
|
|
Surveyor
|
Centaur engine,
|
a hydrogen
|
|
model
|
and send Surveyor
|
peroxide leak
|
|
|
model to a target
|
and too little
|
|
|
location simulating
|
remained to
|
|
|
the Moon
|
power tank boost pumps
|
Flight
|
Date
|
Mission
|
Objective Outcome
|
AC-9
|
Oct. 26,
|
R&D,
|
Demonstrate Successful
|
|
1966
|
two-burn,
|
restart capability,
|
|
|
separable
|
send Surveyor
|
|
|
Surveyor
|
model on
|
|
|
model
|
simulated
trajectory to Moon
|
difficulties are summarized in table 5.1, beginning with Atlas – Centaur 2 (AC-2).38
Data from instrumentation on the insulation panels over the liquid-hydrogen tank on AC-2 showed conclusively that the design for the panels used on AC-1 was not adequate. Engineers designed thicker panels with heavier reinforcement, increasing their weight 188 by almost 800 pounds. This made it all the more important to jet – Chapter 5 tison them at about 180 seconds after launch to get rid of the unwanted weight. A minor redesign fixed the problem with the drive – shaft that failed on AC-3. To fix the problem on AC-4 with liquid hydrogen moving away from the bottom of the tank where the fuel had to exit, however, required investigation and multiple modifications. A slosh baffle in the liquid-hydrogen tank helped limit movement of the fuel away from the tank bottom. Screens in the ducts bringing bleed-off hydrogen gas back to the tank reduced energy that could disturb the liquid. On the coasting portion of AC-4’s orbit, liquid hydrogen had gotten into a vent intended to exhaust gaseous hydrogen, thereby releasing pressure from boil-off. The liquid exiting into the vacuum of space created a sideward thrust that tumbled the Centaur and Surveyor models. Fixing this problem required a complete redesign of the venting system.
A further change increased thrust in both the yaw – and pitch – control engines as well as those that settled liquid hydrogen in the bottom of the tank during coast. The added thrust in both types of engines helped keep the Centaur on course and hold the easily displaced liquid hydrogen in the bottom of its tank. Fortunately, these changes were unnecessary before the launch of AC-5 but were implemented for AC-8, which also incorporated the uprated RL10A – 3-3 engine with slightly greater specific impulse from a larger expansion ratio for the exhaust nozzle and an increased chamber pressure.39
Meanwhile, in response to the explosion on AC-5, engineers locked the Atlas valves in the open position. AC-6 amounted to a semioperational flight. The Surveyor model went to the coordinates in space it was intended to reach (simulating travel to the Moon) even without a trajectory correction in midcourse. With AC-7 shifted to a later launch and AC-8 having problems with hydrogen peroxide rather than liquid hydrogen, the Atlas-Centaur combination was ready for operational use, although there would be one more research-and-development flight sandwiched between launches of operational spacecraft (AC-9; see table 5.1). Atlas – Centaur performed satisfactorily on all of the Surveyor launches, although two of the spacecraft had problems. But five of the seven missions were successful, providing more than 87,000 photographs and much scientific information for Apollo landings and lunar studies. Surveyors 1, 2, and 4 all used single-burn operations by Centaur, but Surveyors 3 and 5-7 employed dual-burn trajectories. On Surveyors 5-7 the Atlases were all SLV-3Cs with longer tanks, hence greater propellant volumes. The SLV-3C flew only 17 missions but was successful on all of them before being replaced by the SLV-3D, used with the advanced Centaur D-1A.40
The D-1A resulted from a NASA decision to upgrade the Centaur, with the Lewis Research Center responsible for overseeing the $40 million improvement program, the central feature of which was a new guidance/control computer, developed at a cost of about $8 million. Among payloads for the Centaur D-1A were Intelsat communications satellites. With the first launch of Intelsat V, having more relay capacity (and weight), on December 6, 1980, the Centaur began to use engines that were adjusted to increase their thrust (per engine) from the original 15,000 to about 16,500 pounds. The 93.75 percent success rate for the 32 SLV-3D/D-1A (and D-1AR) launches showed that Silverstein’s insistence on extended testing and detailed oversight had paid off.41
During the early 1980s, General Dynamics converted to new versions of Atlas and Centaur. The Atlas G added 81 inches to the length of the propellant tanks, and Pratt & Whitney made several changes to the Centaur engines, including removal of the boost pump, for a significant weight savings. There was no change in the RL10’s thrust, but further modification shifted from hydrogen peroxide to the more stable hydrazine for the attitude-control and propellant-settling engines. This made the RL10A-3-3A a substantially different machine than its predecessor, the RL10A-3-3.42
As of early 1991, the Centaur had had a 95 percent success rate on 76 flights. This included 42 successes in a row for Centaur D-1
and D-1A between 1971 and 1984. The vehicle, as well as its Atlas booster, would continue to evolve into the 21st century, with the successful launch of an Atlas V featuring a Russian RD-180 engine and a Centaur with a single RL10 engine, signifying both the end of the cold war and the continuing evolution of the technology. Meanwhile, development of the Centaur had led to the use of liquid-hydrogen technology both on upper stages of the Saturn launch vehicle and on the Space Shuttle. Despite a difficult start and continuing challenges, the Centaur had made major contributions to U. S. launch-vehicle technology.43