Campaign objectives:
The 1978 Venus campaign objectives were to repeat the resounding successes of the Venera 9 and 10 landers with new instruments to analyze both the atmosphere and surface. The 1976-77 opportunity was skipped in order to build the new7 apparatus, and a launch in 1978 dictated a much higher arrival velocity at Venus than in 1975. The larger propellant load required for the longer orbit insertion burn was unable to be accommodated together with the mass of the new instruments, so the carrier was downgraded to a flyby role. A positive outcome wras that a flyby spacecraft would remain in view7 of the lander for longer in order to relay data from the surface. Both of the previous landers had still been operating when their orbiters flew7 below their horizons. The new7 lander investigations featured a high resolution color camera and an experiment to drill into the surface. The descent investigations included new7 experiments to study the chemical composition of the atmosphere, the nature of the clouds, and any electrical activity in the atmosphere. The flyby instrumentation was reduced in order to maximize the mass available for the descent and surface science.
Spacecraft launched
|
|
First spacecraft:
|
Venera 11 (4V-1 No.360)
|
Mission Type:
|
Venus Flyby; Lander
|
Country; Builder:
|
USSR NPO-Lavoehkin
|
Launch Vehicle:
|
Proton – K
|
Launch Date; Time:
|
September 9* 1978 at 03:25:39 UT (Baikonur)
|
Encounter Date / Time:
|
December 25, 1978
|
Outcome:
|
Successful.
|
Second spacecraft:
|
Venera 12 (4V-1 No.361)
|
Mis si on Type:
|
Venus Flyby/Lander
|
Country! Builder:
|
USSR NPO-Lavoehkin
|
Launch Vehicle:
|
Proton – K
|
Launch Date ‘: 7 ime:
|
September 14. 1978 at 02:25:13 UT (Baikonur)
|
Encounter Date/ Time:
|
December 21, 1978
|
Outcome:
|
Successful.
|
Spacecraft:
Although assigned only to a flyby role the Venera 11 and 12 spacecraft were almost identical to their or biter predecessors, but the lander relay was increased to 3 к bits/s per channel. After releasing the entry system 2 days prior to arriving at the planet, Venera 11 (and all later flyby spacecraft) made a deflection maneuver to establish a flyby w7hich would enable it to relay to Earth for longer than w^as possible using an
orbitcr. The spacecraft were identical and the landers had the same configuration as their recent predecessors but the floodlights were deleted and the camera lens cap was redesigned. The complexity and mass of the parachute system was reduced to accommodate more instruments. Only a single supersonic braking parachute was used instead of a sequence of two, and only one main parachute was used instead of a system of three. Some of the instruments were modified and new ones were added, in some cases being installed on the shock absorbing impact ring. All landers from now through to Vega 2 carried a technology experiment consisting of a set of small solar cells arranged around the lander ring.
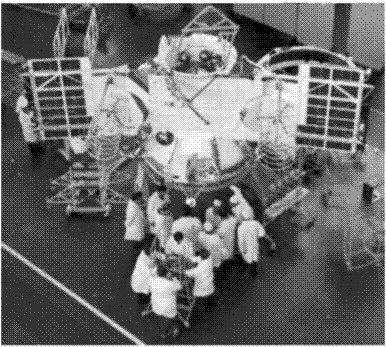 |
4,450 kg (Venera 11) 4,461 kg (Venera 12) 2,127 kg 1,600 kg
731 kg
Payload:
Flyby spacecraft:
1. Extreme-ultraviolet (30 to 166 nm) spectrometer (France)
2. Magnetometer
3. Plasma spectrometer
4. Solar wind detectors
5. High energy particle detectors
6. KONUS gamma-ray burst detector
7. SNEG gamma-ray burst detectors (France-USSR)
KONUS was an interplanetary cruise experiment to try and identify the source of mysterious astronomical gamma-ray bursts by having the two spacecraft coordinate with the Prognoz satellite in Earth orbit to triangulate on individual bursts. SNEG was an instrument complementary to KONUS, built in cooperation with the French. And a new French-built extreme-ultraviolet spectrometer covered the spectral lines of atomic hydrogen, helium, oxygen and other elements that it was thought might be present in the exosphere of Venus. The solar wind detector was a hemispherical proton telescope, and the high energy particle experiments used four semiconductor counters, two gas-discharge counters and four scintillation counters.
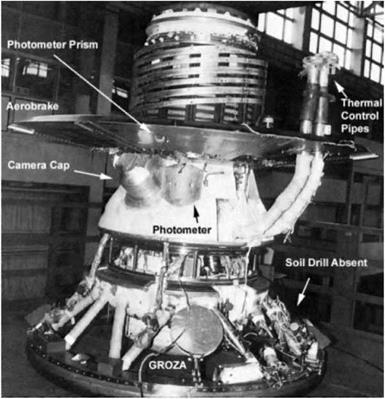
Figure 15.2 Venera 11 lander. The midriff panel is removed providing a view into the interior, and some of the instruments are labeled (from Don Mitchell).
|
Lander:
Entry and descent:
1. Scanning spectrophotometer (0.43 to 1.17 microns)
2. Mass spectrometer Гог atmospheric composition
3. Gas chromatograph for atmospheric composition
4. Nephelometer for aerosols of about 1 micron in size
5. X-ray fluorescence spectrometer for elemental composition of aerosols
6. Accelerometers for atmospheric structure from 105 to 70 km
7. Temperature and pressure sensors for 50 km to the surface
8. GROZA radio sensor at 8 to 95 kHz for electrical and acoustic activity
9. Doppler experiment for wind and turbulence
The experiments for determining the light scattering properties of the atmosphere were modified for a higher spectral resolution. The angular scattering nephelometers were deleted because they had satisfactorily measured the particle size and refractive index when carried on the Venera 9 and 10 landers. Instead, only the back scattering nephelometers w ere retained to examine the spatial uniformity of the cloud layers in different regions of the planet. The scanning spectrophotometer was improved for a higher spectral resolution. Every 10 seconds it measured radiation coming from the zenith using a ramp interference filter at a resolution of about 20 nm continuously over the range 430 to IT70 nm, and the angular distribution of radiation (a full 360 degrees) in the vertical plane in the bands 0.4 to 0.6, 0.6 to 0.8, 0.8 to 1.3. and 1.1 to
1.6 microns using a rotating prism mounted on the aerobrake. The temperatures and pressures were measured by a suite of four thermometers and three barometers, flic monopole radio-frequency mass spectrometer of Venera 9 and 10 w as replaced by a Bennett radio-frequency design and the inlet system modified to prevent it becoming clogged by cloud particles which might then contaminate the atmospheric readings. The microscopic leak admitting the atmosphere to the instrument w7as replaced by a piezoelectric valve that would open a relatively large hole for a very short time in order to admit a pulse of atmosphere into a long sample tube which w? ould trap cloud particles. In addition the instrument was not to be operated until the lander wras at about 25 km. well below the aerosols. The apparatus w^as pumped down between atmospheric readings to purge the sample. Two other new atmospheric composition experiments were included. The gas chromatograph used neon to carry atmospheric samples through columns of porous materials and a Penning ionization detector. It had one column 2 meters long that was optimized for water, carbon dioxide and the compounds hydrogen sulfide, carbonyl sulfide, and sulfur dioxide; a second column 2.5 meters long for the volatile gases helium, molecular hydrogen, argon, molecular oxygen, molecular nitrogen, krypton, methane and carbon monoxide; and a third column just 1 meter long specifically for argon. An x-ray fluorescence spectrometer used gamma rays to excite the emission of x-rays from cloud particles collected on a cellulose acetate filter by drawing atmospheric gas through the instrument, thereby measuring the elemental composition of the aerosols.
The GROZA experiment comprised an acoustic detector and an electromagnetic wave detector using loop antennas with four narrow-band receivers at 10, 18, 36 and 80 kHz and a wide-band receiver over the range 8 to 95 kHz. This was to start at an altitude of 60 km and operate down to and on the surfaec. The electromagnetic wave detector was to register radio bursts from lightning and the acoustic signals could be interpreted in terms of thunder, wind speed past the lander during the descent and, while on the ground, perhaps even seismic quakes.
Surface:
1. Panoramic two-camera color imaging system
2. Soil drill with x-ray fluorescence spectrometer analysis system
3. Rotating conical soil penetrometer (PrOP-V)
The panoramic camera system had been improved by adding clear, red, green and blue filters for three-color imaging, and by increasing the image quality from 128 x 512 pixels at 6 bit encoding to 252 x 1,024 pixels at 9 bit encoding and 1 bit parity. It was capable of resolving detail as fine as 4 or 5 mm at a range of 1.5 meters. The transmission bandwidth had been increased by a factor of twelve, one reason for this being the Kvant-D upgrade to the Soviet communications facilities; in particular the introduction of 70 meter antennas at Yevpatoria and Ussuriisk. The increase in the transmission rate from the surface of Venus from 256 bits, s to 3,000 bits/s enabled a color panorama to be sent in 14 minutes, as against 30 minutes for a lower resolution black-and-white panorama previously.
The gamma-rav soil analysis instrument inside the Venera 8, 9 and 10 landers was replaced by a superior instrument. A drill mounted on the shock-absorbing impact ring w as to core a sample of the surface and then pass it through a series of pressure – reduction stages to the x-ray fluorescence spectrometer carried inside the lander. The penetrometer was on a deployable arm and reported its results on a dial that was to be read by the cameras.
1ission description:
Venera 11 lander:
Venera 11 was launched on September 9, 1978, and made midcourse corrections on September 16 and December 17. After the entry capsule was released on December 23 the spacecraft made the deflection burn in order to perform a flyby of the planet at the desired relay communications altitude, and on December 25 the entry system hit the atmosphere at 11.2 km/s. After a 1 hour descent the lander touched down at a speed of 7 to 8 m/s on the day-side at 14 S 299 E. It w as 03:24 UT, 11:10 Venus solar time, and the solar zenith angle was 17 degrees. The lander transmitted from the surface for 95 minutes before the relay spacecraft flew over the horizon after 110 minutes, so none of the transmission was lost.
figure 15.3 Venera 11 and Venera 12 encounter design, showing entry capsule targeting followed by flyby vehicle deflection and lander relay communications.
Venera 12 lander:
Venera 12 was launched on September 14, 1978, pursued a faster trajectory with midcourse corrections on September 21 and December 14, and arrived ahead of its partner. It released its entry system on December 19. This entered the atmosphere on December 21 at a velocity of 11.2 km/s. The parachute was jettisoned at 49 km and after a 1 hour descent the lander touched down at about 8 m/s on the day-side at 7°S 294°E. It was 03:30 UT, 11:16 Venus solar lime, and the solar zenith angle was 20 degrees. Unlike Venera 11, it kicked up a cloud of dust that took about 25 seconds to settle. Both landers encountered an unexplained anomaly at an altitude of 25 km, where instrument readings went off-scale and there was an electrical discharge from the vehicle. This lander transmitted from the surface for 110 minutes until the flyby spacecraft passed below the horizon. It is therefore not known when it finally ceased to function.
Venera 11 and 12 flyby spacecraft:
After the deflection maneuver, each spacecraft Hew by Venus at a range of about
35,0 km and relayed the data from its lander to Earth throughout the descent and then during the period of surface activity. The last reports from the flyby spacecraft were in January 1980 for Venera 11 and March 1980 for Venera 12.
Results:
Venera 11 and 12 decent measurements:
The landers inferred atmospheric density from accelerometer data over the altitude range 100 to 65 km and then directly measured atmospheric temperature and pressure from 61 km down to the surface. Opacity was measured from 64 km to the surface, the chemical composition of aerosols from 64 km to 49 km, aerosol scattering from 51 km down to the surface, and thunderstorm activity from 60 km down to the surface. The gas chromatograph analyzed nine atmospheric samples from 42 km to the surface. The new mass spectrometer measured atmospheric composition from 23 km to 1 km. Wind velocities were measured from about 23 km down to the surface, and altitude profiles of horizontal wind speed and direction were obtained from Doppler data.
The spectrophotometer produced the first realistic water vapor profile, identifying water vapor as the second most important greenhouse gas in the atmosphere (after carbon dioxide). The contemporary analysis indicated a profile that decreased from 200 ppm at the cloud base to 20 ppm at the surface, but a re-analysis many years later obtained a better fit for the Venera 11,12 and 14 spectrophotometer data using a constant mixing ratio for water vapor of about 30 ppm from 50 km to the surface. The mass spectrometers on these missions reported values as high as 0.5% at 44 km and 0.1% at 24 km; these are much larger mixing ratios for water vapor than were obtained from the spectrophotometer and other remote spectral measurements from Hanh, and are considered suspect.
The mass spectrometer results from Venera 11 and 12 obtained by the analysis of 176 complete spectra of 22 samples were reported as:
carbon dioxide
molecular nitrogen
argon
neon
krypton with isotopic ratios as follows:
0.
0112 ± 0.0002 1.19 ± 0.07 0.197 ± 0.002
The gas chromatograph made eight measurements betw een 42 km and the surface with the following results:
2.5
+ 0.3 %
25 to 100 ppm
40+10 ppm
130 + 35 ppm
28 + 7 ppm (low altitudes)
less than 20 ppm
krypton
hydrogen sulfide carbonyl sulfide
The x-ray fluorescence spectrometer on Venera 12 measured cloud particles from 64 km to 49 km and was then overcome by the high temporal tires. It missed sulfur (< 0.1 mg/m3). but found chlorine (0.43 + 0.06 mg/nri) in cloud particulates. The chlorine was suspected to be a non-volatile compound such as aluminum chloride at the time, but it was not specifically identified. The large amount of chlorine relative to sulfur was incompatible with the theory that the clouds were composed of sulfuric acid droplets, but these anomalous data were corrected by the Venera 14 mission.
Both Venera 11 and 12 detected a large number of electromagnetic pulses in the descent from 32 to 2 km similar to those produced by distant lightning Hashes on Harth. The activity was more intense on Venera 11 than Venera 12, and diminished in intensity towards the surface. No such pulses were detected by Venera 11 after it touched down, but one large burst was noted by Venera 12 while on the surface. The microphones were saturated by aerodynamic noise during the descent and detected no thunder on the surface, but they did pick up sounds issued by the instruments and surface activities.
As with Venera 9 and 10. the light scattering data indicated clouds with a base at an altitude of 47 km, and a much lower loading of aerosols below that. Venera 11 and 12 found the atmosphere to be generally free of aerosols below ^30 km. The nephelometer on Venera 11 measured cloud particles throughout the descent and its results confirmed the uniformity of the cloud layers as reported by Venera 9 and 10. The base cloud layer was located between 51 and 48 km, with a mist below that. The nephelomeler on Venera 12 did nol function correctly. It was confirmed that only about 3 to 6% of the sunlight reaches the surface. Intense Rayleigh scattering in the dense atmosphere gives poor visibility. Above several kilometers altitude the surface must be invisible. At ground level the horizon will be visible, but the detail of the landscape must fade quickly into an orange haze. The Sun is not visible as a disk, merely a uniformly lit hazy sky.
Venera 11 and 12 surface measurements:
The temperature at the Venera 11 landing site was 458 + 5 C and the pressure was 91 + 2 bar. There was no surface imaging because the lens covers would not open. These had been redesigned after the problems w ith one camera on each of Venera 9 and 10, but with disastrous results. The transmit ted pictures were uniformly black. The soil drill collected a sample, but it was not properly delivered to the instrument container and no soil analysis was accomplished.
The temperature at the Venera 12 site was 468 + 5 C and the pressure was 92 + 2 bar. The fact that this suffered exactly the same camera and soil analysis experiment failures as its partner implied a systematic design flaw. Vibrations while descending broke the sample transfer system on the drill and no soil analysis was possible. The soil penetrometers also failed on both landers.
The surface experiments were an almost total failure on both landers. It is possible
that they suffered rough landings which damaged the instruments that were mounted on the impact ring. The lack of results was very disappointing, hut in typical Soviet fashion this spurred the engineers on to succeed at the next flight opportunity.
Venera 11 and 12 flyby spacecraft:
The ultraviolet spectrometer detected Lyman-alpha emissions from hydrogen atoms and 584 angstrom (He-I) emissions from helium atoms. These provided exospheric temperatures and number densities. Time profiles for 143 gamma-ray bursts were obtained by Venera 11 and 12 and the results triangulated with an identical detector on Prognoz 7 in harth orbit. On Kebruary 13 and March 17, 1980, Venera 12 used its extreme-ultraviolet spectrometer to observe Comet Bradfield.