Campaign objectives:
With the survival of the Venera 8 capsule on the surface of Venus in 1972, the 3MV spacecraft had reached the limit of its capability and the Soviets were ready for the next step. They now had enough data on the atmosphere of Venus and conditions at the surface to design a very capable lander that included sophisticated imaging and surface science instruments. The challenge was to enable this apparatus to operate in such harsh conditions. Also, the new heavy Proton-launched Mars spacecraft had proved itself in 1971 with the Mars 2 and 3 missions. Both orbiters were successful, and the Mars 3 lander was successfully delivered to the surface. This orbiter served as the basis for designing the Venus spacecraft. However, the entry vehicle had to be completely redesigned. For the first time since their initial launch to Venus in 1961, the Soviets skipped a Venus opportunity in October 1973 while they w’orked on their new spacecraft.
The main difference between the heavy Proton-launched spacecraft for Mars and for Venus was the entry system. A vehicle to enter the rarefied atmosphere of Mars needs a broad conical aerobrake lor rapid deceleration in the upper atmosphere and large robust parachutes to slow to a safe speed before reaching the surface. The thick atmosphere of Venus, on the other hand, is much more forgiving and permits the use
of a simpler entry system. The new system for Venus was a hollow spherical vessel that contained the heavy lander and its parachute system. The previous probes had revealed the atmosphere to be so thick that to reach the surface in a reasonable time the new system w as designed to jettison its parachute high in the atmosphere and let the lander fall using an aerobrake. and since the free-fall velocity at the surface was slow enough for the impact to be survivable there w as no requirement for a terminal retro-rocket.
Spacecraft launched
|
|
First spacecraft:
|
Venera 9 (4V-1 Ко.660)
|
Mission Type:
|
Venus Orbiter/Lander
|
Conn try j Builder:
|
USSR /NPO-Lavochkin
|
Launch Vehicle:
|
Proton-K
|
Launch Daie] І ime:
|
June 8, 1975 at 02:38:00 UT (Baikonur)
|
Lncoun ter Date/ 7 і me:
|
October 22. 1975
|
Or hi ter Ter minuted:
|
March 22, 1976
|
Outcome:
|
Successful.
|
Second spacecraft:
|
Venera 10 (4V-1 Ко.661)
|
Mission Type:
|
Venus Or bi ter / Lander
|
Conn try j Builder:
|
USSR, NPO-Lavochkin
|
Launch Vehicle:
|
Proton-K
|
Launch Date ‘: 7 ime:
|
June 14, 1975 at 03:00:31 UT (Baikonur)
|
Lncoun ter Date/ 7 ime:
|
October 25. 1975
|
Orbi ter Terminated:
|
March 22, 1976
|
Outcome:
|
Successful.
|
There were minor modifications to the spacecraft, including changes in the size and position of the solar panels, the thermal systems, and increased reliability. One key change was to replace the dircet-to-Harth communications system of the lander with a system to relay via the or biter, which greatly improved the data rate from the lander. The mission plan for Venus differed from that for Mars. The orbiter lander w’as first targeted at the atmospheric entry point, rather than at the orbital insertion point. Several days from Venus the passive entry system w’as released. Immediately afterwards, the spacecraft made a deflection maneuver for the orbital insertion point. The timing was such that by the time the lander started to transmit, the spacecraft would have just completed its orbit insertion burn and have a line of sight to relay the transmission.
The principal scientific goal of the lander w^as to obtain the first panoramic image on the surface of Venus. This determined the minimum time that the lander must be able to operate on the surface and also the data rate of the relay through the orbi ter. These new* capabilities, and the large mass available on the lander, meant a number of instruments that had never been flown before could be carried Гог descent science. These included instruments to measure the vertical structure of aerosols w’ithin and under clouds, the vertical and spectral distribution of solar flux penetrating through
the clouds for several look angles, chemical and isotopic analysis of the atmosphere, and direct measurements of the winds at the surface. To undertake the first science from orbit, the spacecraft had experiments to report upon the plasma environment around the planet and its atmospheric structure, upper cloud layers, and outgoing thermal radiation.
Early on, consideration had been given to using a flyby spacecraft to support the lander mission but NPO-Lavochkin and IK I argued hard for the orbiter in order to obtain the additional and original science that only an orbiter could provide. And, of course, being first to place a spacecraft in orbit around the planet would be another significant first achievement in space exploration.
Spacecraft:
As the first of another generation of spacecraft, Venera 9 and 10 were five times heavier than their predecessors and were launched by the more powerful four-stage Proton-K. This launcher was introduced in 1969 for the Ye-8 lunar missions and then used for the M-69, M-71 and M-73 campaigns. These new spacecraft consisted of an orbiter with the entry system strapped on top, inside of w hich w as the lander. The new entry system and lander were extensively tested in wind tunnels and by airdrops.
The two spacecraft for this opportunity were essentially identical, but Venera 10 was slightly heavier and required a larger fuel load for its longer orbit insertion burn.
kg (Venera 9) 5,033 kg (Venera 10) kg (Venera 9) 1,159 kg (Venera 10) kg (Venera 9) 2,314 kg (Venera 10) kg
The orbiter was based on the M-71 spacecraft. The IJDMH and nitrogen tetroxide tanks formed a cylindrical body. At 110 cm in diameter, this was narrower than the 180 cm Mars version and it was also 1 meter shorter. Below’ was the KTDU-425A restartable rocket engine which could be throttled between 9,856 and 18,890 N for a total of 560 seconds. The avionics and science instruments were in a pressurized toroidal module with a diameter of 2.35 meters that was attached at the base of the cylinder, with the gimbaled engine nozzle protruding through the donut. Navigation optics attached to the exterior of the instrument module included a number of solar sensors mounted in a linear cluster, bordered either side by duplicate down-looking telescopic Canopus sensors. The Earth sensor was installed in such a way as to point in the same direction as the parabolic high gain antenna. With the entry system on top, the spacecraft was 2.8 meters tall.
Tw o 1.25 x 2.1 meter solar arrays extended from opposite sides of the cylinder with an overall span of 6.7 meters. These supported cold gas attitude control jets, the
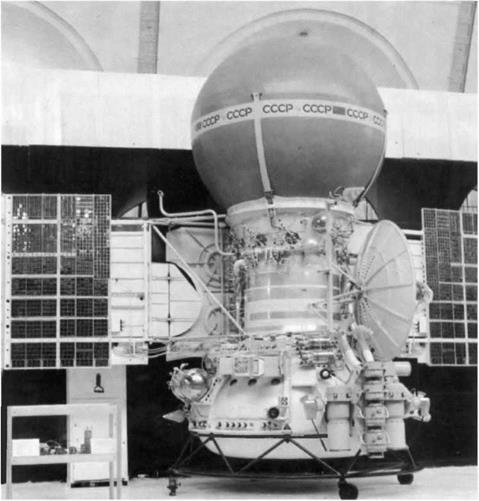
Figure 14.1 Venera 9 spacecraft.
|
magnetometer booms, and a relay antenna for the entry system and lander. Also on the side of the cylinder were thermal control gas radiators and tanks which contained nitrogen at 350 bar for the attitude control system. During the interplanetary cruise, louvers on the entry shell provided passive thermal control of the entry system. Communications from the entry system and lander were received by the orbiter and relayed to Earth. There was a 1.6 meter diameter parabolic high gain antenna on the side of the cylinder for communicating with Earth on decimeter and centimeter bands. Six omnidirectional helical antennas were attached near the parabolic antenna, four for Earth and two for the lander. The command uplink was by the helical antennas at 769 MHz. There was a 16 megabyte magnetic tape system for data storage. The downlink to Earth was phase modulated PCM at 3 kbits/s via the
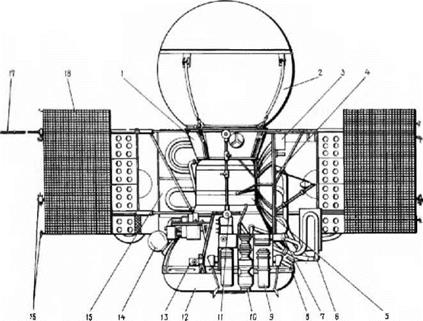
Figure 14.2 Venera 9 and Venera 10 spacecraft: 1. Orhiter bus; 2. Descent capsule; 3. Science instruments; 4. High-gain antenna; 5. Propellant tank; 6. Thermal control pipes; 7. Earth sensor; 8. Science instruments; 9. Canopus sensor; 10. Sun sensor; 11. Omnidirectional antenna; 12. Science instrument module; 13. Science instruments; 14. Altitude control gas tank; IS. Thermal control radiator; 16. Attitude control jets; 17. Magnetometer; 18. Solar panels.
|
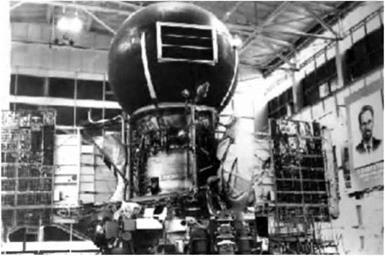
Figure 14.3 Venera 9 in test at NPO-Lavoehkin. The shutters on the entry vehicle are for thermal control during flight.
|
high gain antenna, or in an emergency at a much slower rate using the helical antennas. Data from the lander was retransmitted through the parabolic antenna to Earth in real-time and also stored on tape for later backup transmission. The spacecraft computers were similar to those carried by the M-71 missions.
As it did for Mars, this design served as the basis for all Venus spacecraft starting in 1975, and the Proton-K became the singular planetary launch vehicle in the Soviet inventory.
Entry system:
The sophisticated new lander was contained within a 2.4 meter diameter spherical entry system, and was deployed after the rate of descent through the atmosphere had been reduced to subsonic. The entry vessel was a simple sphere covered by ablative material that consisted of asbestos composite over honeycomb, and it was stabilized during entry by placing the center of mass towards leading side. The entry angle was shallower than for the 3MV capsules, reducing the peak load from around 450 G to a more modest 150 to 180 G. After entry, the sphere would split into hemispheres, releasing the lander and its parachute system.
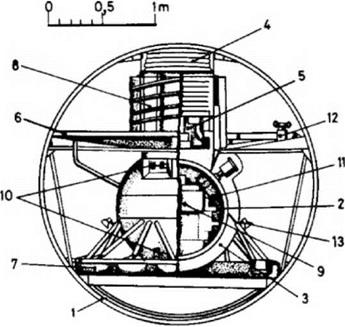
Figure 14.4 Venera 9 and Venera 10 entry capsule (from Space Travel Encyclopedia): 1. Heat shield; 2. Lander instrument compartment; 3. Lander insulation; 4. Parachute; 5. Descent instruments; 6. Descent brake; 7. Landing ring shock absorber; 8. Transmitter helical antenna; 9. Electronics; 10. Science instruments; 11. Panoramic camera; 12. Anemometer; 13. Illumination lamp.
|
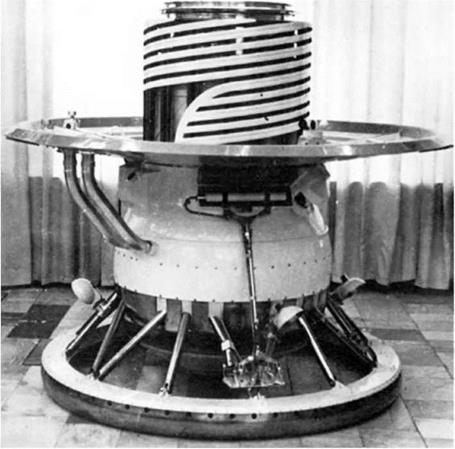
Figure 14.5 Venera 9 lander with shock absorbing lander ring, spherical pressurized instrument compartment, and upper disk drag brake with cylindrical wound antenna ‘top hat’. A camera pod can be seen at right under the disk brake next to the ‘paint – roller’ gamma-ray densitometer folded up against the sphere. The spectrophotometer housing is under the disk at the left. Floodlights are attached to the shock absorber struts to illuminate the fields of view of the two cameras. An anemometer for surface winds is mounted on the top of the disk at left. The outer insulating layers are removed. The two severed pipes on the left are for pre-cooling by the orbiter before separation.
|
Lander:
The lander was 2 meters in height, which was much larger than the 3MV capsules, and capable of carrying more scientific instruments. Previous Venera probes were limited to a transmission rate of 1 bit/s by their direct communications link to Earth. The lander was battery powered and transmitted to the orbiter through two VHF channels at 256 bits/s for relay to Earth using the high gain antenna.
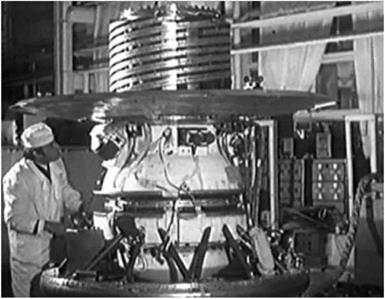
Figure 14.6 Venera 9 lander during tests. The central band segment of the pressure vessel
is removed for access. The engineer is looking at a camera.
|
The lander was basically a hermetically sealed titanium spherical pressure vessel 80 cm in diameter, containing most of the instrumentation and electronics. This was affixed to a ring-shaped landing cushion by a set of shock absorbers. Above was a disk-shaped aero brake 2.1 meters diameter, to slow the rate of descent during free – fall. This disk also acted as a reflector for the cylindrically wound omnidirectional antenna above. Inside this 80 cm diameter 40 cm tall cylinder were the parachutes and some of the descent instruments. The sphere consisted of several sections bolted together with gold wire seals. It was surrounded with a 12 cm layer of honeycomb insulating material and a thin surface of titanium. The inside of the sphere was also lined with a polyurethane foam insulating material. The thermal design was similar to the earlier landers. The lander was pre-chilled to -10°C by cold air from the main spacecraft via two pipes through the entry vessel. A lithium nitrate trihydrate phase – change material which melted at 33°C absorbed heal that penetrated the insulation, and a gas circulation system distributed it evenly. These measures, and the life of the batteries, allowed operation for about an hour after landing.
Entry, descent and, landing:
The midcourse maneuvers were to target the spacecraft for the entry point. The entry system w’ould be released 2 days from Venus to make a ballistic approach and enter the atmosphere at 10.7 km/s at an angle in the range 18 to 23 degrees. Six seconds later it would experience the peak deceleration of 170 G. After 20 seconds, having slowed to 250 m/s and under a 2 G load at an altitude of about 65 km. the small pilot
parachute would deploy with a ripcord to draw out the 2.8 meter drogue parachute. The spherical shell would then split into hemispheres and the drogue would pull the upper hemisphere and attached lander away from the lower hemisphere, at the same time deploying a second braking parachute. After 11 seconds, by now at an altitude of 60 to 62 km and descending at 50 m/s, the upper hemisphere would release the lander, in the process extracting the three 4.3 meter diameter main parachutes from the cylindrical section on top of the lander.
Once on its main parachutes, the lander would activate its instruments. It would spend about 20 minutes descending through the cloud layer at a rate of about 50 m/s. On reaching an altitude of 50 km, the lander would shed its parachutes and spend the next 55 minutes free falling, with the drag of the disk-shaped aerobrake slowing its rate of descent as it penetrated the thicker air close to the surface. This strategy was selected to minimize heat inflow during descent, and hence extend the lifetime of the lander on the surface. The terminal velocity on reaching the surface would be 7 m/’s, and the impact would be cushioned by the compressible, metal annular landing ring.
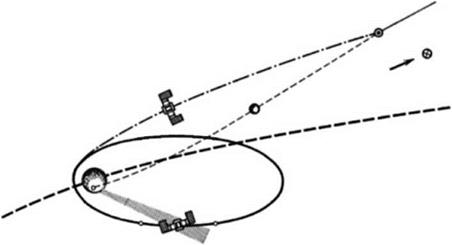
Figure 14.7 Approach geometry and relay operations for the lander. The entire vehicle is initially targeted for the impact point on the sunlit side, out of view of Earth. Two days out, the entry vehicle is released and the orbiter makes a deflection maneuver to place it in position for an orbit insertion burn before the entry vehicle arrives. Shortly after orbit insertion, the orbiter is in position above the landing site for relay operations during entry, descent and landing.
|
Payloads:
Orbiter:
1. Panoramic ultraviolet cloud cameras, 345 to 380 nm and 355 to 445 nm
2. Cloud infrared spectrometer (1.6 to 2.8 microns)
3. Cloud thermal infrared radiometer (8 to 28 microns)
4. Cloud ultraviolet imaging spectrometer (352 and 345 nm) [USSR-France]
5. Cloud photopolarimeters (335 to 800 nm)
6. Lyman-alpha H/D photometer
7. Airglow spectrometer (300 to 800 nm).
8. Triaxial magnetometer
9. Plasma electrostatic analyzer
10. Charged particle traps
11. Cherenkov energetic particle detectors
12. Centimeter and decimeter radio occultation experiment
13. Bistatic 32 cm radar mapping experiment
The two cloud cameras were the same as the linear scanning photometer cameras of the Mars 4 and 5 orbiters. providing cross-track scanning of 30 degrees and using the motion of the spacecraft to scan along the orbital track. The Venus cameras used violet and ultraviolet filters, scanned 500 cycles/line at 2 lines/second. Images were usually transmitted at 256 pixels/line with 6 bits/pixel. Panoramas were typically
6,0 pixels in length. For a periapsis at about 5,000 km the resolution at the cloud tops was on the order of 6 to 30 km.
Between them, the spectrometers and photometers could make measurements of the clouds throughout the ultraviolet, visible and infrared parts of the spectrum. The photopolarimeters were an improved form of those of the M-71 and M-73 missions, with design help from the French. The cloud infrared spectrometer used a circular – ramp interference filter and made high resolution spatial scans across the planet. The thermal infrared instrument used two horn radiometers for bands at 8 to 13 microns and 18 to 28 microns, both of which were relatively transparent in an atmosphere of carbon dioxide. The French-built cloud ultraviolet imaging spectrometer measured spatial profiles across the planet at two wavelengths with a resolution of 16 seconds of arc. The particle detectors included low energy electron, proton and alpha particle sensors, three semiconductor counters, two gas discharge counters, and a Cherenkov detector.
Lander:
Entry and descent:
1. Broadband photometer with three visible and two infrared channels for radiation flux
2. Narrow-band infrared photometer with three channels near 0.8 microns for radiation flux ratios in water, carbon dioxide and background bands
3. Back scatter and multi-angle nephelomctcrs at 0.92 microns for light scattering between the altitudes of 63 and 18 km
4. Pressure and temperature measurements from 62 km to the surface
5. Accelerometers for atmospheric structure between 110 and 76 km
6. Mass spectrometer for atmospheric composition from 63 to 34 km altitude
7. Doppler experiment for wind and turbulence
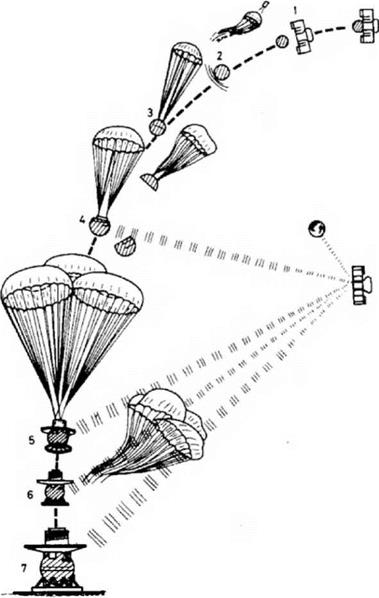
Figure 14.8 Venera 9 and Venera 10 descent sequence (from Space Travel Encyclopedia): 1. Capsule release two days before entry; 2. Atmospheric entry, 170 G max; 3. Pilot chute withdraws first parachute; 4. First chute pulls top away and deploys second braking chute. Radio and instruments activated; 5. Main chutes open at 62 km, bottom shell jettisoned. Science investigations conducted during 20 min descent through clouds; 6. Lander released at 50 km altitude; 7. Lander on the surface 55 min later.
|
|
In comparison to Venera 8, the new photometers were greatly improved and more complex. Both up welling and down welling integrated radiation was measured in the range 0.440 to 1.160 microns using green, yellow, red. IR1. and IR2 glass filters for five wavelength hands with widths of 0.1 to 0.3 microns. This was complemented by an near-infrared photometer operating in three channels, one centered on the carbon dioxide band at 0.78 microns, another on the water band at 0.82 microns, and a third background channel at 0.80 microns, with each band being only 0.005 microns wide. Both back scattering and angular scattering nephelometers were carried. These were new, measuring how7 the atmosphere scattered light from a pulsed light source. This information could be used to infer the size distribution, refractive index, and density of cloud droplets. The sensors for the photometers and nephelometer w ere mounted in the external environment, had their own thermal protection, and were linked by fiber optics to the instruments inside. The mass spectrometer was a radio-frequency monopole unit with a pressure regulator designed for input pressures of 0.1 to 10 bar. The Doppler experiment was facilitated by an ultra-stable master oscillator for the transmitter.
Surface:
1. Panoramic imaging system, two cameras with floodlights
2. Surface wind rotary anemometer
3. Gamma-ray spectrometer (uranium, potassium, and thorium) for surface rocks
4. Gamma-ray densitometer
The scanning photometer imaging camera w as similar to that carried by the M-71 landers and had a mass of 5.8 kg. There were tw o, in sealed insulated containers on either side of the lander just beneath the disk of the aero brake to give a vantage point 90 cm off the ground. The rotational axis of the mirror system was tilted from the landers vertical by 50 degrees in order that the center of the image was the surface directly in front of the camera at a distance of 1.5 to 2 meters, with the field of view extending 90 degrees to either side in order to include a small section of the horizon. The cameras peered through 1 cm thick cylindrical quartz windows using a lens to compensate for refraction and provide a total angular field of 40 x 180 degrees. Each 128 x 512 panorama consisted of a 115 x 512 image. w7ith the first 13 bits of each line containing a calibration pattern. Each measurement consisted of a 6 bit picture element and 1 bit for parity checking. The quality of the imagery w7as limited by the projected 30 minute surface lifetime and the transmission rate of 256 bits s. To send a panorama at 3.5 seconds per line would require 30 minutes. The panoramas w7ere to be transmitted simultaneously on separate VHF channels. Because scientist were worried after Venera 8 that the illumination at the surface would be very weak, each camera was provided with a 10,000 lux floodlight system with tw7o lamps in order to ensure that there would be sufficient light to acquire an image.
The deployable densitometer had a cesium-137 irradiation source and detectors to measure the gamma rays reflected back by the environment. During the descent this measured atmospheric scattering. Immediately after landing it deployed a 4 x 36 cm
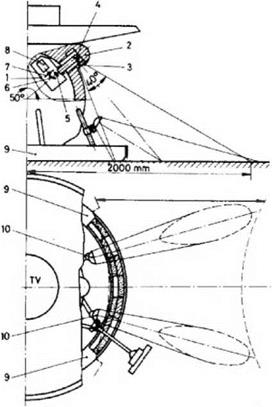
Figure 14.9 Television system mounted on Venera 9 and Venera 10 landers showing (a) camera and illuminator mounting, line-of-site FOV, and (b) imaging panorama and illuminator footprints (from Space Travel Encyclopedia): 1. Panoramic camera; 2. Insulation; 3. Camera port; 4. Scanning тіїтог; S. Lens; 6. Mirror; 7. Pressure diaphragm; 8. Photometer; 9. Landing ring; 10. Illumination lamp.
|
‘paint roller’ on the surface in order to measure soil scattering. In addition, a sodium iodide gamma-ray spectrometer similar to that of Venera 8 was carried inside the sphere for measurement of potassium, uranium and thorium soil abundances. Two anemometers were mounted on the upper side of the aerobrake disk.
Mission description:
Venera 9 orbitev:
Venera 9 was launched on June 8, 1975. It maneuvered on June 16 and October 15 to align its trajectory with the desired entry point in the atmosphere of Venus. After releasing its entry system on October 20, it performed a 247.3 m/s deflection burn to head for the orbit insertion point, where on October 22 it made a 922.7 m/s burn and inserted itself into an orbit with a period of 48.30 hours. The relay of the transmission from the entry system and lander followed immediately thereafter. This was the first spacecraft to enter orbit around the planet. The initial orbit was 1.500 x 111,700 km inclined at 34.17 degrees. This was changed to 1,300 x 112,200 km and finally to 1,547 x 112,144 km at 34.15 degrees. The orbiter conducted 3 months of scientific observations which were terminated by the failure of its transmitter.
Venera 9 lander:
The entry system penetrated the atmosphere of Venus at a speed of 10.7 к m/s and at an angle of 20.5 degrees. At 05:13 UT on October 22 the lander touched down at a speed of 7 to 8 m/s on the day-side of the planet at 31.01 N 291.64 E, where it was 13:12 Venus solar time and the solar zenith angle was 33 degrees. The site was on a slope of 15 to 20 degrees and the lander was tilted a further 10 to 15 degrees by the uneven, rocky surface. It immediately began its surface activities, relaying its data to Earth via the orbiter until that flew out of range 53 minutes later, by which time the temperatures inside the lander had risen to 60 C.
Venera 10 orbiter:
After launch on June 14. 1975, Venera 10 flew7 almost the same route as Venera 9. making trajectory corrections on June 21 and October 18, releasing its entry system on October 23 and then making a 242.2 m s deflection burn. On October 25 it made a 976.5 m/s insertion burn. Its initial orbit was 1,500 x 114.000 km inclined at 29.50 degrees with a period of 49.38 hours. This was later changed to 1,651 x 113,923 km at 29.10 degrees. After relaying the transmission from the entry system and lander, the orbiter began its scientific observations. It succumbed 3 months later to the same problem as disabled its partner.
Venera 10 lander:
The entry system penetrated the atmosphere of Venus at an angle of 22.5 degrees at 01:02 UT on October 25. The lander touched down at 02:17 UT at a speed of about 8 m/s at 15.42 N 291.5UE, about 2,200 km from w here Venera 9 landed. It was on the day-side, at 13:42 Venus solar time with a solar zenith angle of 27 degrees. The surface was fairly level but the lander was perched on a rocky mass that tilted it at about 8 degrees. The lander was still transmitting when the orbiter flew out of range, curtailing the relay operation after 65 minutes.
Results:
Venera 9 lander:
Entry and decent science
The Venera 9 lander inferred atmospheric density from accelerometer data between the altitudes 110 and 76 km. It directly measured atmospheric temperature, pressure, composition and light levels from 62 km to the surface. Light scattering data from the nephelometer. together with the photometer data, indicated clouds with a base at an alii Hide of 49 to 48 km and a lower loading of aerosols extending down to about 25 + 5 km. The clouds were similar to a light fog. with much smaller drop size than normal for Earth and a visibility of several kilometers. Distinct layers were detected at altitudes of 60 to 57 km, 57 to 52 km and 52 to 49 km. The refractivity index was measured to be as high as 1.46; much higher than for water ice and consistent with sulfuric acid droplets. The cloud particles all scattered light but there was absorption in the blue and this, along with heavy Rayleigh scattering, led to increasing orange color with depth. The atmosphere below 25 km appeared to be free of aerosols. Red light was found to reach further towards the surface than blue, shifting the spectrum towards longer w avelengths, producing both orange colored skies and, by reflection, an orange tinted surface. Doppler data provided altitude profiles of horizontal wind speed and direction.
The detailed chemical composition measurements attempted by the first landers of this type gave poor results. The mass spectrometers did not function properly due to inappropriate cleaning procedures prior to launch and apparent clogging of the inlet system by cloud particles. The mixing ratio of molecular nitrogen to carbon dioxide was determined. Argon was deteeted in the atmosphere. A large ratio of argon-36 to argon-40 was measured and confirmed by later missions, but went unreported owing to mistrust of the instrument. Near-infrared photometer results for the water vapor mixing ratio proved to be spurious when spectral measurements were conducted on later missions.
Surface science
The photometers detected dust raised by the landing, but this quickly settled. The surface conditions were 455 + S C and 85 + 3 bar, and there w? as a light w? ind of 0.4 to 0.7 m/s.
Only one 180 degree panorama was taken because the cover for the other camera failed to deploy. This back-and-white image was the first picture from the surface of another planet. It showed a level landscape with a variety of flat, apparently young angular rocks without much erosion. Л portion of the image extended to the horizon, and there w’as no indication of dust in the atmosphere. The illumination was similar to Earth mid-latitudes on a cloudy summer day, and the light scattering did not east shadows. The floodlights for the camera were not triggered on. They were eliminated from subsequent missions. The visibility was a pleasant surprise to the scientists who, after reviewing Venera 8, had predicted a dark, murky and dusty atmosphere in which only the near field would be available for inspection.
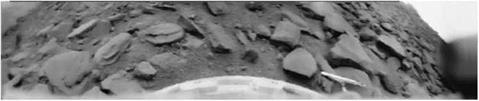
Figure 14.10 Venera 9 lander 180 degree panorama (processing by Ted Stryk).
|
The indistinctness and apparent nearness of the horizon in all of the images from the Venera landers were due to the high refractivity of the dense atmosphere which made Venus appear to be a small-diameter spherical body with a horizon much less than 1 km away. The phenomenon is similar to a terrestrial mirage and is probably a function of the observer’s height above the ground.
The gamma-ray composition analysis of the surface material measured potassium, uranium and thorium abundances more typical of terrestrial basalt than meteorites. The fact that the surface rocks differed from primitive meteorites in a way consistent with trends observed in terrestrial rocks indicated that Venus must have been thermal differentiated into a core, mantle and crust. The reflectivity of the surface in five wavelengths was consistent with material of a basaltic composition. The penetrometer indicated a rock density of 2.7 to 2.9 g/’cc.
Venera 10 lander:
Entry and descent science
The Venera 10 lander inferred atmospheric density from accelerometer data between the altitudes 110 and 63 km. ft directly measured atmospheric temperature, pressure, composition and light levels from 62 km to the surface, and structure, microphysical properties and composition of the clouds. The three distinct cloud layers observed by Venera 9 were confirmed. Doppler data profiled the horizontal wind speed and direction during the descent, and then an anemometer measured wind velocity on the surface. As the results were generally in agreement with Venera 9, some conclusions could be drawn on atmospheric convective stability and turbulence. The profile of temperature and pressure showed 33 bar and 158"’C at 42 km, 37 bar and 363°C at 15 km, and 91+3 bar and 464 + 5°C at the surface.
Surface science
As in the case of Venera 9, one of the camera covers failed to deploy and this lander also only provided a single 180 degree black-and-white panoramic image. It showed a surface that was smoother with large, more eroded pancake rocks interspersed with lava or other weathered rocks. The horizon was visible and there was no evidence of dust in the atmosphere. As with Venera 9, the photometers detected some dust raised on touchdown which quickly settled. A surface albedo of 0.06 was derived from the imaging and photometers on both landers.
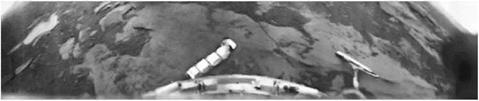
figure 14.11 Venera 10 lander 180 degree panorama (processing by Ted Stryk).
|
The surface winds were light at 0.8 to 1.3 m/s. The gamma-ray results and surface reflectivity were suggestive of a basaltic composition. Apparently both landers came down on young volcanic shield structures with lavas close in composition to the tholeiitie basalts that emerge from oceanic spreading ridges on Earth. The penetrometer indicated a surface density of 2.7 to 2.9 g/cc, just as at the Venera 9 site. The surface of Venus appeared to be harder than the Moon or Mars.
Venera 9 and 10 orbitersi
The panoramic cameras returned 1,200 km long images taken using several different filters to distinguish cloud structures and some surface features, although the latter were poorly defined. The results included imagery of the clouds in the ultraviolet, infrared radiometry, photometry, spectrometry of both day and night sides, photo- polarimetry, radio occultation and plasma data. Orbital data suggested a cloud base at an altitude of 30 to 35 km with three distinct layers. The orbiters obtained data on the clouds above 64 km, which is the altitude at which the descent data started. The day-time temperature of the upper cloud was -35°C. warming by about 10 degrees at night. The night-time atmosphere was found to glow in the visible spectral range in bands that later investigation established to be a molecular oxygen band system that is not excited in the Earth’s atmosphere owing to its lower concentration of carbon dioxide.
The airglow spectrometer on the Venera 9 orbiter found optical evidence of night-side lightning, but Venera 10 did not. Reflection spectra of clouds in the infrared at 1.7 to 2.8 microns measured the aerosol scale height near their upper boundary, and infrared wide band radiometry in the range 8 to 28 microns prompted the conclusion that outgoing radiation is systematically stronger on the night-side than the day-side.
The dual frequency radio occult ations at wavelengths of 8 and 32 cm gave a set of temperature and pressure profiles for altitudes in the range 40 to 80 km that revealed details of the night-side ionosphere and the existence of a large diurnal variation of ionospheric electron density. The bistatic radar experiment mapped fifty-five strips of the surface 100 to 200 km wide by 400 to 1,200 km long. Early analysis provided onc-dimensional terrain profiles at a resolution of 20 to 80 km. Later processing of the Venera 10 data produced a two-dimensional local topography for live regions at a resolution of 5 to 20 km.
Measurements of the scattering of solar Lyman-alpha radiation by the hydrogen corona that surrounds Venus, including its line width, gave an estimate of 450°C for
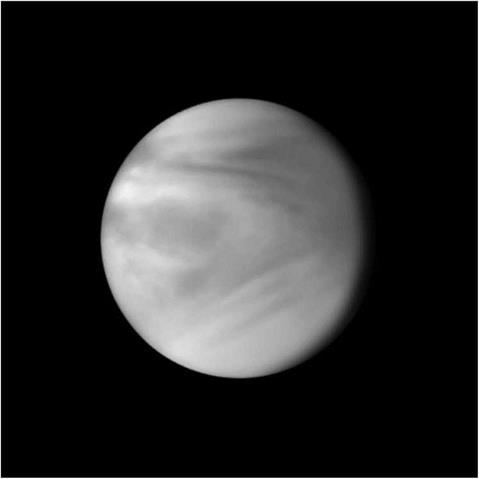
Figure 14.12 Mosaic of the planet from images by the Venera 9 orbiter (courtesy Ted Strvk).
|
the temperature of the atmosphere at the exobase. Many features of how the solar wind interacts with the ionosphere were measured. No intrinsic planetary magnetic field was detected. Nonetheless, the interaction of the solar wind with the ionosphere created a magnetic plasma tail.
As the first spacecraft to enter Venus orbit, Venera 9 and 10 were able to provide the first long-term survey of the Venusian atmosphere with a comprehensive battery of scientific instrumentation. Their landers performed marvelously, returning the first pictures of the surface of the planet. These missions began what became an unbroken string of successes running to Venera 16 in 1983 and ending with the two Vega missions that made flybys in 1985.