As the 1970s came to an end, the U. S. military fleet of high-performance fighter aircraft had been transformed from departure-prone designs to new configurations with outstanding stability and departure resistance at high angles of attack. Thanks to the national research and development efforts of industry and Government following the Dayton symposium in 1971, the F-14, F-15, F-16, and F/A-18 demonstrated that the peril of high-angle-of-attack departure exhibited by the previous generation of fighters was no longer a critical concern. Rather, the pilot could exploit high angles of attack under certain tactical conditions without fear of nose slice or pitch-up. At air shows and public demonstrations, the new "supermaneuverable” fighters wowed the crowds with high – angle-of-attack flybys, and more importantly, the high-alpha capabilities provided pilots with new options for air combat. High-angle-of-attack
technology had progressed from concerns over stall characteristics to demonstrated spin resistance and was moving into a focus on poststall agility and precision maneuverability.
Reflecting on the advances in high-angle-of-attack technology of the 1970s and concepts yet to be developed, technical managers at Langley, Dryden, and Ames began to advocate for a cohesive, integrated research program focused on technologies and innovative ideas. The Agency was in an excellent position to initiate such a program thanks to the unique ground – and flight-testing capabilities that had been developed and the expertise that had been gathered by interactions of the NASA researchers with the real-world challenges of specific aircraft programs. At Langley, for example, researchers had been intimately involved in high-angle-of – attack/departure/spin activities in the development of all the new fighters and had accumulated in-depth knowledge of the characteristics of the configurations, including aerodynamics, flight control architecture, and handling characteristics at high angles of attack. Technical expertise and facilities at Langley included subscale static and dynamic free – flight model wind tunnel testing, advanced control-law synthesis, and computational aerodynamics. In addition, extensive peer contacts had been made within industry teams and DOD aircraft development offices.
At Dryden, the world-class flight-test facilities and technical expertise for high-performance fighter aircraft had been continually demonstrated in highly successful flight-test programs in which potentially hazardous testing had been handled in a professional manner. The Dryden staff was famous for its can-do attitude and accomplishments, including the conception, development, and routine operation of experimental aircraft; advanced flight instrumentation; and data extraction techniques.
Meanwhile, at Ames, the aeronautical research staff had aggressively led developments in high-performance computing facilities and computational aerodynamics. Computational fluid dynamics (CFD) codes developed at Ames and Langley had shown powerful analysis capability during applications to traditional aerodynamic predictions such as cruise performance and the analysis of flow-field phenomena. In addition to computational expertise, Ames had extensive wind tunnel facilities, including the huge 80-by 120-Foot Tunnel, which had the capability of testing a full-scale fighter aircraft as large as the F/A-18.
From the perspective of the three technical managers, the time was right to bring together the NASA capabilities into a focused program directed toward some of the more critical challenges in
high-angle-of-attack technology.[1308] The research program that evolved from the planning meetings grew into one of the more remarkable efforts ever undertaken by NASA. The planning, advocacy, and conduct of the program was initiated at the grassroots level and was managed in a most remarkable manner for the duration of the program. Within NASA’s aeronautics activities, the program brought an enthusiastic environment of cooperation—not competition—that fostered a deep commitment to team spirit and accomplishments so badly needed in research endeavors. The personal satisfaction of the participants was widely known, and the program has become a model for NASA intercenter relationships and joint programs.[1309]
The first task in planning the program was to identify major technical issues facing the high-angle-of-attack community. Foremost among these was the understanding, prediction, and control of aerodynamic phenomena at high-angle-of-attack conditions, especially for aircraft configurations with strong vortical flows. Achieving this goal involved detailed studies of separated flow characteristics; measurement of static and dynamic phenomena in ground-test facilities as well as flight; calibration of flow predictions from CFD methodology, wind tunnels, and flight; and the development of CFD codes for high-angle-of-attack conditions. In addition, the analysis and prediction of aerodynamic phenomena associated with structural fatigue issues for vertical tails immersed in violently fluctuating separated flows at high-angle-of-attack conditions became a major element in the program.
The second research thrust in the proposed program was directed toward an exciting new technology that offered unprecedented levels of controllability at high angles of attack—thrust vectoring. The thrustvectoring concept had been developed in early rocket control applications by placing vanes in the exhaust of the rocket vehicle, and extensive NASA-industry-DOD studies had been conducted to develop movable nozzle vectoring concepts for aircraft applications. The introduction of the superb fighters of the 1970s had demonstrated a new level of design achievement in stability at high angles of attack, but another nemesis remained—inadequate control at high-angle-of-attack conditions at
which conventional aerodynamic control surfaces lose effectiveness because of separated flow. The problem was particularly critical in the lack of ability to create crisp, precise roll control for "nose pointing” at high angles of attack. For such conditions, the ability to roll is dependent on providing high levels of yaw control, which creates sideslip and rolling motion because of dihedral effect. Unfortunately, conventional rudders mounted on vertical tails become ineffective at high angles of attack.
During the early 1980s, researchers at the Navy David Taylor Research Center pursued the application of simple jet-exit vanes to the F-14 for improved yaw control.[1310] Teaming with Langley in a joint study in the Langley Differential Maneuvering Simulator, the researchers found that the increased yaw control provided by the vanes resulted in a dramatic improvement in high-angle-of-attack maneuverability and dominance in simulated close-in air combat. Inspired by these results, Langley researchers evaluated the effectiveness of similar vanes on a variety of configurations during free-flight model testing in the Langley Full Scale Tunnel. Following investigations of modified models of the F-16, F/A-18, X-29, and X-31, the researchers concluded that thrust vectoring in yaw provided unprecedented levels of maneuverability and control at high angles of attack. In addition, providing feedback from flight sensors to the vane control system enhanced dynamic stability for the test conditions.
Another technology that had matured to the point of research applications was the control of strong vortical flow shed from the long pointed forebodies of contemporary fighters at high angles of attack. As previously mentioned, Dryden and the Air Force Flight Dynamics Laboratory had conducted a joint program to evaluate the effects of blowing on the nose of the X-29A for enhanced control. Competing concepts for vortical flow control had also received attention during NASA and industry research programs, including investigations at Langley of deflectable forebody strakes that could be used to control flow separation on the forebody for enhanced yaw control.
Perhaps the most contentious issue in planning the integrated NASA high-angle-of-attack program was whether a research aircraft was required and, if so, which aircraft would make the best testbed for research studies. Following prolonged discussions (the Ames
representative did not initially endorse the concept of flight-testing), the planning team agreed that flight-testing was mandatory for the program to be relevant, coordinated, and focused. Consideration was given to the F-15, F-16, X-29, and F/A-18 as potential testbeds, and after discussions, the team unanimously chose the F/A-18, for several reasons. The earlier Navy F/A-18 development program had included extensive support from Langley; therefore, its characteristics were well known to NASA (especially aerodynamic and aeroelastic phenomena, such as vortical flow and vertical tail buffet). During spin-testing for the development program, the aircraft had displayed reliable, stall-free engine operations at high angles of attack and excellent spin recovery characteristics. The F/A-18 was equipped with an advanced digital flight control system that offered the potential for modifications for research flight tests. Finally, the aircraft exhibited a remarkably high-angle-of-attack capability (up to 60 degrees in trimmed low-speed flight)—ideal for aerodynamic tests at extreme angles of attack.
The intercenter planning team presented its integrated research program plan to NASA Headquarters, seeking approval to pursue the acquisition of an F/A-18 from the Navy and for program go-ahead. After Agency approval, the Navy transferred the preproduction F/A-18A Ship 6, which had been used for spin testing at Patuxent River, MD, to NASA Dryden, where it arrived in October 1984. This particular F/A-18A had been stripped of several major airframe and instrument components following the completion of its spin program at Patuxent River, but it was still equipped with a multi-million-dollar emergency spin recovery parachute system and a programmable digital flight control computer ideally suited to NASA’s research interests. The derelict aircraft was shipped overland to Dryden and reassembled by a team of NASA and Navy technicians into a unique high-angle-of-attack research airplane known as the F/A-18A High-Alpha Research Vehicle (HARV).[1311] The HARV was equipped with several unique research systems, including flow visualization equipment, a thrust-vectoring system using external postexit vanes around axisymmetric nozzles, and deployable nose strakes on a modified fuselage of forebody. Additional aircraft systems included extensive instrumentation, integrated flight research controls with special flight control hardware and software for the thrust-
vectoring system, interface controls for the forebody strakes, and safety backup systems including a spin recovery parachute.
The High-Angle-of-Attack Technology program (HATP) was funded and managed under an arrangement that was different from other NASA programs but was extremely efficient and productive. Headquarters provided program management oversight, but recommendations for day – to-day technical planning, distribution of funds, and technical thrusts were provided by an intercenter steering committee consisting of members from each of the participating Centers. In recognition of its technical expertise and accomplishments in high-angle-of-attack technology, Langley was designated the technology lead Center. Dryden was designated the lead Center for flight research and operations of the HARV, and Ames and Langley shared the technical leadership for CFD and experimental aerodynamics. In subsequent years, the NASA Lewis Research Center (now the NASA Glenn Research Center) joined the HATP for experiments on engine inlet aerodynamics for high-angle-of-attack conditions. The HATP included aerodynamics, flight controls, handling qualities, stability and control, propulsion, structures, and thrust vectoring.[1312]
The HATP program was conducted in three sequential phases, centering on high-angle-of-attack aerodynamic studies (1987-1989), evaluation of thrust vectoring effects on maneuverability (1990-1994), and forebody flow control (1995-1996), with 383 research flights. In the first activities, aerodynamic characteristics obtained from flight-test results for the baseline HARV (no vectoring) were correlated with wind tunnel and CFD predictions, with emphasis on flow separation predictions and vortical flow behavior on the fuselage forebody and wing-body leading-edge extension (LEX).[1313]
The first HARV research flight was April 17, 1987. Flown in its baseline configuration, the HARV provided maximum angles of attack on the order of 55 degrees, limited by aerodynamic control. At the time the flight studies were conducted, CFD had not yet been applied to real
aircraft shapes at high angles of attack. Rather, researchers had used computational methods to predict flow over simple shapes such as prolate spheroids, and the computation of flow fields, streamlines, and separation phenomena for a modern fighter was extremely challenging. Many leaders in the NASA and industry CFD communities were pessimistic regarding the success of such a venture at the time.
The experimental wind tunnel community was also facing issues on how (or whether) to modify models to better simulate high-angle – of-attack aerodynamics at flight values of Reynolds numbers and to understand the basic characteristics of vortical flows and techniques for the prediction of flow interactions with aircraft structures. Using a highly innovative, Dryden-developed propylene glycol monomethyl ether (PGME) dye flow-visualization technique that emitted colored dye tracers from ports for visualization of surface flows over the HARV forebody and LEX, the team was able to directly compare results, analyze separation phenomena, and modify CFD codes for a valid prediction of the observed on-surface flow characteristics. The ports for the PGME were later modified for pressure instrumentation to provide even more detailed information on flow fields. Additional instrumentation for aerodynamic measurements was initially provided by a nose boom, but evidence of aerodynamic interference from the nose boom caused the team to remove it and replace the boom with wingtip air-data probes. A rotating, foldout flow rake was also used to measure vortical flows shed by the LEX surfaces.[1314]
The results of the HATP flight – and ground-based aerodynamic studies provided a detailed perspective of the relative accuracy of computational flow dynamics and wind tunnel testing techniques to predict critical flow phenomena such as surface pressures, separation contours, vortex interaction patterns, and laminar separation bubbles.[1315] The scope of correlation included assessments of the impact of Mach and Reynolds numbers on forebody and LEX vortexes as observed in flight with the HARV, the Langley 7- by 10-Foot High-Speed Tunnel, the Langley
30- by 60-Foot Tunnel, the Navy David Taylor Research Center 7- by 10-Foot Transonic Tunnel, and the Ames 80- by 120-Foot Wind Tunnel.
A wide variety of subscale models of the HARV configuration was tested in the various wind tunnels, and a full-scale F/A-18 aircraft was used for testing in the Ames 80- by 120-Foot Tunnel. The test article was an ex-Blue Angel flight demonstrator, whose life had been exceeded,
that had been bailed to NASA for the tests. When the tunnel tests were conducted in 1991 and 1993, the aircraft had both engines, flowthrough inlets, and the wingtip missile launchers removed.
Using extensive instrumentation that had been carefully coordinated between ground and flight researchers gathered an unprecedented wealth of detail on aerodynamic characteristics of a modern fighter at high angles of attack. The effort was successful particularly because it had been planned with common instrumentation locations for pressure ports and flow visualization stations between wind tunnel tests and the flight article. More importantly, the high value of the data obtained was the result of one of the most successful aspects of the program—close communications and working relationships between the flight, wind tunnel, and CFD technical communities.
As NASA neared the end of the aerodynamic phase of testing for the HARV, growing concerns over buffeting of the vertical tail surfaces for military fleet F/A-18 aircraft led the Navy and McDonnell-Douglas to develop vertical longitudinal fences on the upper surfaces of the LEX to
extend the service life of the tails of fleet F/A-18s. Although the fences were not installed on HARV during the early aerodynamic studies, they were added during the second and third phases of the program, when extensive wind tunnel and HARV flight studies of the tail buffet phenomenon were conducted. Resulting data were transmitted to the appropriate industry and service organizations for analysis of the F/A-18 specific phenomena as well as for other twin-tail fighter aircraft.
As the second phase of the HATP began, Dryden accepted major program responsibilities for the implementation of a relatively simple and cheap thrust-vectoring system for the HARV aircraft. The objective of NASA’s research was not to develop a production-type thrustvectoring engine/nozzle system, but rather to evaluate the impact of vectoring for high-angle-of-attack maneuvers, assess control-law requirements for high-angle-of-attack applications, and use the control augmentation provided by vectoring to stabilize the aircraft at extreme angles of attack for additional aerodynamic studies. With this philosophy in mind, the program contracted with McDonnell-Douglas to modify the HARV with deflectable external vanes mounted behind the aircraft’s two F-404 engines, similar in many respects to the installations used by the Navy F-14 mentioned earlier and the Rockwell X-31 research aircraft.
For the installation, the exhaust nozzle divergent flaps were removed from the engines and replaced with a set of three vanes for each engine, thereby providing both pitch and yaw vectoring capability. The research teams at Dryden and Langley thoroughly studied the specific vane configuration, structural design, and control system modifications required for the project. The scope of activities included measurements of thrust – vane effectiveness for many powered model configurations at Langley, simulator studies of the effectiveness of vectoring on maneuverability and controllability at Langley, and hardware and software development— as well as the integration, checkout, and operations of the system—at Dryden. The implementation of the HARV thrust-vectoring hardware and software modifications proved to be relatively difficult, requiring the NASA research team to participate in the final design of the thrustvectoring system. The HARV vectoring system followed the HATP objective of providing thrust-vectoring research capability at minimal cost through external airframe modifications rather than a new production – type vectoring engine. With the massive external thrust-vectoring vane actuation system and the emergency spin recovery parachute system both mounted on the rear of the aircraft and necessary ballast added
 |
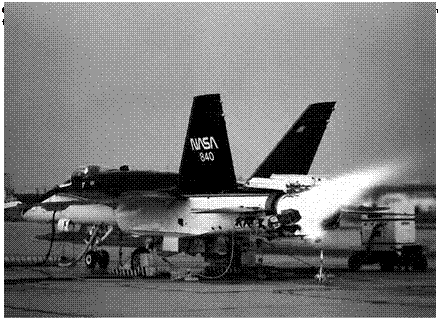 |
to the nose of the aircraft to maintain balance, the weight of the HARV was increased by about 4,000 pounds.
In the thrust-vectoring phase of the HATP project, the conventional flight control system of the HARV was modified to include a research flight control system (RFCS) to influence control laws. The conventional F/A-18 control laws were used for takeoff, for landing, and as a backup in case of failure of the RFCS, whereas the second set of control laws were for high-angle-of-attack research flights. The design and implementation of the RFCS system was one of the more complex changes to the F/A-18 digital flight control system undertaken at that time.
First flight of the HARV with vectoring engaged occurred in July 1991, a few weeks after the X-31 research aircraft demonstrated pitchvectoring capability at Edwards, but the HARV conducted the first multiaxis vectoring flights shortly thereafter. Research flight-testing of the HARV equipped with thrust vectoring vividly demonstrated the anticipated benefits at high angles of attack that had been predicted by earlier free – flight model tests and piloted simulator studies. The precision and angular rates available to the pilot were remarkable, and the enhanced stability and control at extreme angles of attack permitted precision aerodynamic
studies that had previously been impossible. Angles of attack as high as 70 degrees were flown with complete control in aerodynamic experiments.
During the late 1980s, three NASA-industry-DOD programs had been initiated to explore thrust-vectoring systems for high-angle-of – attack conditions. Each program had different objectives and focused on separate technologies. NASA’s HARV aircraft was designed to evaluate fundamental thrust-vectoring system control-law synthesis and use vectoring to stabilize the aircraft at high angles of attack for aerodynamic experiments. The DARPA X-31A aircraft was conceived to demonstrate enhanced fighter maneuverability at poststall angles of attack under simulated tactical conditions. In addition, the Air Force F-16 Variable – Stability In-Flight Simulator Test Aircraft (VISTA) was modified into the F-16 Multi-Axis Thrust Vectoring (MATV) project with an objective of demonstrating the effectiveness of a production-type thrust-vectoring system. All three programs had different goals, and the three research aircraft underwent flight-testing at Edwards in the same time period.
The HATP participants conceived, developed, and assessed several control-law schemes, which included special configurations for longitudinal control at high angles of attack, lateral and directional control mixing strategies, automatic spin prevention, and spin recovery modes. Seventy-five spin attempts (at low power conditions) resulted in 70 fully developed spins with satisfactory recoveries, and the emergency spin recovery parachute was never fired in flight.
As the HARV conducted its thrust-vectoring research program, a critical issue emerged within the advanced fighter design community. With new configurations under consideration having extreme angle-of – attack capability and reduced longitudinal stability for performance and maneuverability enhancements, the issue of providing sufficient nose – down control effectiveness for recovery from high-angle-of-attack excursions became significant. NASA-DOD technical meetings had been held to discuss studies to assess the adequacy of theoretical and wind tunnel predictions, and it appeared that using the HARV flight capability with thrust vectoring would provide highly desirable data for design criteria for future fighters. In view of the urgency of the situation, Langley led a HATP element known as High-Alpha Nosedown Guidelines (HANG), which included extensive simulator studies and flights with the HARV.[1316]
Although the main objective of the HARV thrust-vectoring experiments was not air-to-air combat maneuvering, Dryden conducted flight tests to provide validation data for a proposed high-angle-of-attack flying qualities requirement MIL-STD-1797A by using basic fighter maneuvers and limited air combat maneuvering. Six NASA research test pilots from Dryden and Langley provided the major expertise and guidance for the HATP simulator and HARV flight-testing. Other guest pilots from NASA, the Navy, the Canadian Air Force, the United Kingdom, McDonnell-Douglas, and Calspan also participated in flight-test evaluations of the HARV vectoring capabilities.
The third and final phase of the HATP was directed to in-flight assessments of the effectiveness of controlling the powerful vortex flows shed by the fuselage forebody for augmentation of yaw control at high angles of attack. Ground-based research in NASA wind tunnels and simulators had indicated that the most effective method for rolling an aircraft about its flight path for nose pointing at high angles of attack was through the use of yaw control. Unfortunately, conventional rudders suffer a severe degradation and control effectiveness at high angles of attack because of the impingement of low-energy stalled flows only vertical tail surfaces. Years of NASA research had demonstrated that the use of deployable fuselage forebody strakes was a potentially viable concept for yaw control augmentation. With a vast amount of wind tunnel data and pilot opinions derived from air combat simulation, the strake concept was ready for realistic evaluations in flight. Once again, the cohesive nature of the HATP was demonstrated when the strake hardware was designed and fabricated on a special F/A-18 forebody radome in machine shops at Langley and the control laws were developed at Langley and delivered to Dryden, where the flight computer interface and instrumentation were accomplished by the Dryden staff. The project, known as actuated nose strakes for enhanced rolling (ANSER), was evaluated independently and in combination with thrust vectoring.[1317]
Implementation of the ANSER concept on the thrust-vectoring – equipped HARV provided three control combinations. The aircraft could be flown with thrust vectoring only, thrust vectoring in longitudinal control with a thrust-vectored and strake-blended mode for lateral control,
and a strake mode with thrust-vectoring control longitudinally and strakes controlling the lateral mode. As was the case for thrust vectoring, the forebody strake flight results demonstrated that a significant enhancement of high-angle-of-attack rolling capability was obtained, particularly at higher subsonic speeds. In fact, at those speeds, the effectiveness of the strakes was comparable to that of thrust vectoring.
Several other subsystems were implemented on the HARV, including an instrumented inlet rake, extensive pressure instrumentation, aero – servoelastic accelerometers, thrust-vectoring vane loads and temperatures, and an emergency power backup system. Notably, although the power backup system was implemented to continue aircraft systems operation in the event of a dual-engine flameout or unrecoverable dualengine stalls, it was removed later in the program when testing showed excellent high-angle-of-attack engine operations. In fact, 383 high-angle – of-attack flights were made without experiencing an engine stall.
Throughout the HATP program, NASA ensured that results were widely disseminated within industry and DOD. Major HATP technical conferences were held, with at least 200 attendees at Langley in 1990, at Dryden in 1992 and 1994, and a wrap-up conference at Langley in 1996.[1318] Hundreds of reports and presentations resulted from the program, and the $74 million (1995 dollars) activity produced cutting-edge technical results that were absorbed into the Nation’s latest aircraft, including the F-22, F-35 and F/A-18E.