Manufacture of the silica tiles was straightforward, at least in its basic steps. The raw material consisted of short lengths of silica fiber of l.0-micron diameter. A measured quantity of fibers, mixed with water, formed a slurry. The water was drained away, and workers added a binder of colloidal silica, then pressed the material into rectangular blocks that were 10 to 20 inches in diameter and more than 6 inches thick. These blocks were the crudest form of LI-900, the basic choice of RSI for the entire Shuttle. They sat for 3 hours to allow the binder to jell, then were dried thoroughly in a microwave oven. The blocks moved through sintering kilns that baked them at 2,375 °F for 2 hours, fusing binder and fibers together. Band saws trimmed distortions from the blocks, which were cut into cubes and then carved into individual tiles using milling machines driven by computer. The programs contained data from Rockwell International on the desired tile dimensions.
Next, the tiles were given a spray-on coating. After being oven-dried, they returned to the kilns for glazing at temperatures of 2,200 °F for 90 minutes. To verify that the tiles had received the proper amount of coating, technicians weighed samples before and after the coating and glazing. The glazed tiles then were made waterproof by vacuum deposition of a silicon compound from Dow Corning while being held in a furnace at 350 °F. These tiles were given finishing touches before being loaded into arrays for final milling.[610]
Although the basic LI-900 material showed its merits during 1972, it was another matter to produce it in quantity, to manufacture tiles that were suitable for operational use, and to provide effective coatings. To avoid having to purify raw fibers from Johns Manville, Lockheed asked that company to find a natural source of silica sand with the necessary purity. The amount needed was small, about 20 truckloads, and was not of great interest to quarry operators. Nevertheless, Johns Manville found a suitable source in Minnesota.
Problems arose when shaping the finished tiles. Initial plans called for a large number of identical flat tiles, varying only in thickness and trimmed to fit at the time of installation. But flat tiles on the curved surface of the Shuttle produced a faceted surface that promoted the onset of turbulence in the airflow, resulting in higher rates of heating. The tiles
then would have had to be thicker, which threatened to add weight. The alternative was an external RSI contour closely matching that of the orbit – er’s outer surface. Lockheed expected to produce 34,000 tiles for each orbiter, grouping most of them in arrays of two dozen or so and machining their back faces, away from the glazed coating, to curves matching the contours of the Shuttle’s aluminum skin. Each of the many thousands of tiles was to be individually numbered, and none had precisely the same dimensions. Instead, each was defined by its own set of dimensions. This cost money, but it saved weight.
Difficulties also arose in the development of coatings. The first good one, LI-0042, was a borosilicate glass that used silicon carbide to enhance its high-temperature thermal emissivity. It dated to the late 1960s; a variant, LI-0050, initially was the choice for operational use. This coating easily withstood the rated temperature of 2,300 °F, but in tests, it persistently developed hairline cracks after 20 to 60 thermal cycles. This was unacceptable; it had to stand up to 100 such cycles. The cracks were too small to see with the unaided eye and did not grow large or cause tile failure. But they would have allowed rainstorms to penetrate the tiles during the weeks that an orbiter was on the ground between missions, with the rain adding to the launch weight. Help came from NASA Ames, where researchers were close to Lockheed, both in their shared interests and in their facilities being only a few miles apart. Howard Goldstein at Ames, a colleague of the branch chief, Howard Larson, set up a task group and brought in a consultant from Stanford University, which also was just up the road. They spent less than $100,000 in direct costs and came up with a new and superior coating called reaction-cured glass. Like LI-0050, it was a borosilicate, consisting of more than 90 percent silica along with boria or boron oxide along with an emittance agent. The agent in LI-0050 had been silicon carbide; the new one was silicon tetraboride, SiB4. During glazing, it reacted with silica in a way that increased the level of boria, which played a critical role in controlling the coating’s thermal expansion. This coating could be glazed at lower temperature than LI-0050 could, reducing the residual stress that led to the cracking. SiB4 oxidized during reentry, but in doing so, it produced boria and silica, the ingredients of the glass coating itself.[611]
The Shuttle’s distinctive mix of black-and-white tiles was all designed as standard LI-900 with its borosilicate coating, but the black ones had SiB4 and the white ones did not. Still, they all lacked structural strength and were brittle. They could not be bonded directly to the orbiter’s aluminum skin, for they would fracture and break because of their inability to follow the flexing of this skin under its loads. Designers therefore placed an intermediate layer between tiles and skin, called a strain isolator pad (SIP). It was a felt made of Nomex nylon from DuPont, which would neither melt nor burn. It had useful elasticity and could stretch in response to Shuttle skin flexing without transmitting excessive strain to the tiles.[612]
Testing of tiles and other thermal-protection components continued through the 1970s, with NASA Ames being particularly active. A particular challenge lay in creating turbulent flows, which demanded close study because they increased the heat-transfer rates many times over. During reentry, hypersonic flow over a wing is laminar near the leading edge, transitioning to turbulence at some distance to the rear. No hypersonic wind tunnel could accommodate anything resembling a full-scale wing, and it took considerable power as well as a strong airflow to produce turbulence in the available facilities. Ames had a 60-megawatt arc-jet, but even that facility could not accomplish this. Ames succeeded in producing such flows by using a 20-megawatt arc-jet that fed its flow into a duct that was 9 inches across and 2 inches deep. The narrow depth gave a compressed flow that readily produced turbulence, while the test chamber was large enough to accommodate panels with size of 8 by 20 inches. This facility supported the study of coatings that led to the use of reaction-cured glass. Tiles of LI-900, 6 inches square and treated with this coating, survived 100 simulated reentries at 2,300 °F in turbulent flow.[613]
The Ames 20-megawatt arc-jet facility made its own contribution in a separate program that improved the basic silica tile. Excessive temperatures caused these tiles to fail by shrinking and becoming denser.
Investigators succeeded in reducing the shrinkage by raising the tile density and adding silicon carbide to the silica, rendering it opaque and reducing internal heat transfer. This led to a new grade of silica RSI with density of 22 lb/ft3 that had greater strength as well as improved thermal performance.[614]
The Ames researchers carried through with this work during 1974 and 1975, with Lockheed taking this material and putting it into production as LI-2200. Its method of manufacture largely followed that of standard LI-900, but whereas that material relied on sintered colloidal silica to bind the fibers together, LI-2200 dispensed with this and depended entirely on fiber-to-fiber sintering. LI-2200 was adopted in 1977 for operational use on the Shuttle, where it found application in specialized areas. These included regions of high concentrated heat near penetrations such as landing-gear doors as well as near interfaces with the carbon-carbon nose cap, where surface temperatures could reach 2,600 °F.[615]
Testing proceeded in four overlapping phases. Material selection ran through 1973 and 1974 into 1975; the work that led to LI-2200 was an example. Material characterization proceeded concurrently and extended midway through 1976. Design development tests covered 1974 through 1977; design verification activity began in 1977 and ran through subsequent years. Materials characterization called for some 10,000 test specimens, with investigators using statistical methods to determine basic material properties. These were not the well-defined properties that engineers find listed in handbooks; they showed ranges of values that often formed a Gaussian distribution, with its bell-shaped curve. This activity addressed such issues as the lifetime of a given material, the effects of changes in processing, or the residual strength after a given number of flights. A related topic was simple but far-reaching: to be able to calculate the minimum tile thickness, at a given location, that would hold the skin temperature below the maximum allowable.[616]
Design development tests used only 350 articles but spanned 4 years, because each of them required close attention. An important goal involved validating the specific engineering solutions to a number
of individual thermal-protection problems. Thus the nose cap and wing leading edges were made of carbon-carbon, in anticipation of their being subjected to the highest temperatures. Their attachments were exercised in structural tests that simulated flight loads up to design limits, with design temperature gradients.
Design development testing also addressed basic questions of the tiles themselves. There were narrow gaps between them, and while Rockwell had ways to fill them, these gap-fillers required their own trials by fire. A related question was frequently asked: What happens if a tile falls off? A test program addressed this and found that in some areas of intense heating, the aluminum skin indeed would burn through. The only way to prevent this was to be sure that the tiles were firmly bonded in place, and this meant all those located in critical areas.[617]
Design verification tests used fewer than 50 articles, but these represented substantial portions of the vehicle. An important test article, evaluated at NASA Johnson, reproduced a wing leading edge and measured 5 by 8 feet. It had two leading-edge panels of carbon-carbon set side by side, a section of wing structure that included its principal spars, and aluminum skin covered with RSI. It could not have been fabricated earlier in the program, for its detailed design drew on lessons from previous tests. It withstood simulated air loads, launch acoustics, and mission-temperature-pressure environments, not once, but many times.[618]
The testing ranged beyond the principal concerns of aerodynamics, heating, and acoustics. There also was concern that meteoroids might not only put craters in the carbon-carbon but also cause it to crack. At NASA Langley, the researcher Donald Humes studied this by shooting small glass and nylon spheres at target samples using a light-gas gun driven by compressed helium. Helium is better than gunpowder, as it can expand at much higher velocities. Humes wrote that carbon-carbon: "does not have the penetration resistance of the metals on a thickness basis, but on a weight basis, that is, mass per unit area required to stop projectiles, it is superior to steel.”[619]
Yet amid the advanced technology of arc-jets, light-gas guns, and hypersonic wind tunnels, one of the most important tests was also one of the simplest. It involved nothing more than taking tiles that were bonded with adhesive to the SIP and the underlying aluminum skin and physically pulling them off.
It was no new thing for people to show concern that the tiles might not stick. In 1974, a researcher at Ames noted that aerodynamic noise was potentially destructive, telling a reporter for Aviation Week that: "We’d hate to shake them all off when we’re leaving.” At NASA Johnson, a 10-MW arc-jet saw extensive use in lost-tile investigations. Tests indicated there was reason to believe that the forces acting to pull off a tile would be as low as 2 psi, just some 70 pounds for a tile measuring 6 by 6 inches square. This was low indeed; the adhesive, SIP, and RSI material all were considerably stronger. The thermal-protection testing therefore had given priority to thermal rather than to mechanical work, essentially taking it for granted that the tiles would stay on. Thus, attachment of the tiles to the Shuttle lacked adequate structural analysis, failing to take into account the peculiarities in the components. For example, the SIP had some fibers oriented perpendicular to the cemented tile undersurface. The tile was made of ceramic fibers, with these fibers concentrating the loads. This meant that the actual stresses they faced were substantially greater than anticipated.[620]
Columbia orbiter OV-102 was the first to receive working tiles. Columbia was also slated to be first into space. It underwent final assembly at the Rockwell plant in Palmdale, CA, during 1978. Checkout of onboard systems began in September, and installation of tiles proceeded concurrently, with Columbia to be rolled out in February 1979. But mounting the tiles was not at all like laying bricks. Measured gaps were to separate them; near the front of the orbiter, they had to be positioned to within 0.17 inches of vertical tolerance to form a smooth surface that
would not trip the airflow into turbulence. This would not have been difficult if the tiles had rested directly on the aluminum skin, but they were separated from that skin by the spongy SIP. The tiles were also fragile. An accidental tap with a wrench, a hard hat, even a key chain could crack the glassy coating. When that happened, the damaged tile had to be removed and the process of installation had to start again with a new one.[621]
The tiles came in arrays, each array numbering about three-dozen tiles. It took 1,092 arrays to cover this orbiter, and NASA reached a high mark when technicians installed 41 of them in a single week. But unfortunate news came midway through 1979 as detailed studies showed that in many areas the combined loads due to aerodynamic pressure, vibration, and acoustics would produce excessively large forces on the tiles. Work to date had treated a 2-psi level as part of normal testing, but now it was clear that only a small proportion of the tiles already installed faced stresses that low. Over 5,000 tiles faced force levels of 8.5 to 13 psi, with 3,000 being in the range of 2 to 6.5 psi. The usefulness of tiles as thermal protection was suddenly in doubt.[622]
What caused this? The fault lay in the nylon felt SIP, which had been modified by "needling” to increase its through-the-thickness tensile strength and elasticity. This was accomplished by punching a barbed needle through the felt fabric, some 1,000 times per square inch, which oriented fiber bundles transversely to the SIP pad. Tensile loads applied across the SIP pad, acting to pull off a tile, were transmitted into the SIP at discrete regions along these transverse fibers. This created localized stress concentrations, where the stresses approached twice the mean value. These local areas failed readily under load, causing the glued bond to break.[623]
There also was a clear need to increase the strength of the tiles’ adhesive bonds. The solution came during October and involved modifying a thin layer at the bottom of each tile to make it denser. The process was called, quite logically, "densification.” It used DuPont’s Ludox
with a silica "slip.” Ludox was colloidal silica stirred into water and stabilized with ammonia; the slip had fine silica particles dispersed in water. The Ludox acted like cement; the slip provided reinforcement, in the manner of sand in concrete. It worked: the densification process clearly restored the lost strength.[624]
By then, Columbia had been moved to the Kennedy Space Center. The work nevertheless went badly during 1979, for as people continued to install new tiles, they found more and more that needed to be removed and replaced. Orderly installation procedures broke down. Rockwell had received the tiles from Lockheed in arrays and had attached them in well-defined sequences. Even so, that work had gone slowly, with 550 tiles in a week being a good job. But now Columbia showed a patchwork of good ones, bad ones, and open areas with no tiles. Each individual tile had been shaped to a predetermined pattern at Lockheed using that firm’s numerically controlled milling machines. But the haphazardness of the layout made it likely that any precut tile would fail to fit into its assigned cavity, leaving too wide a gap with the adjacent ones.
Many tiles therefore were installed one by one, in a time-consuming process that fitted two into place and then carefully measured space for a third, designing it to fill the space between them. The measurements went to Sunnyvale, CA, where Lockheed carved that tile to its unique specification and shipped it to the Kennedy Space Center (KSC). Hence, each person took as long as 3 weeks to install just 4 tiles. Densification also took time; a tile removed from Columbia for rework needed 2 weeks until it was ready for reinstallation.[625]
How could these problems have been avoided? They all stemmed from the fact that the tile work was well advanced before NASA learned that the tile-SIP-adhesive bonds had less strength than the Agency needed. The analysis that disclosed the strength requirements was neither costly nor demanding; it might readily have been in hand during 1976 or 1977. Had this happened, Lockheed could have begun shipping densified tiles at an early date. Their development and installation would have occurred within the normal flow of the Shuttle program, with the change amounting perhaps to little more than an engineering detail.
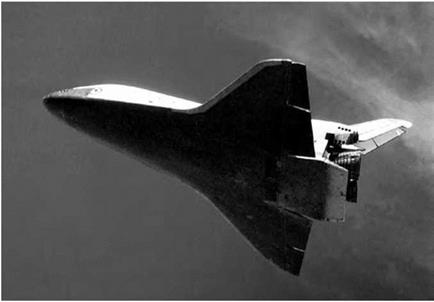
The Space Shuttle Columbia descends to land at Edwards following its hypersonic reentry from orbit in April 1981. NASA.
|
The reason this did not happen was far-reaching, for it stemmed from the basic nature of the program. The Shuttle effort followed "concurrent development,” with design, manufacture, and testing proceeding in parallel rather than in sequence. This approach carried risk, but the Air Force had used it with success during the 1960s. It allowed new technologies to enter service at the earliest possible date. But within the Shuttle program, funds were tight. Managers had to allocate their budgets adroitly, setting priorities and deferring what they could put off. To do this properly was a high art, calling for much experience and judgment, for program executives had to be able to conclude that the low-priority action items would contain no unpleasant surprises. The calculation of tile strength requirements was low on the action list because it appeared unnecessary; there was good reason to believe that the tiles would face nothing worse than 2 psi. Had this been true, and had the main engines been ready, Columbia might have flown by mid-1980. It did not fly until April 1981, and, in this sense, tile problems brought a delay of close to 1 year.
The delay in carrying through the tile-strength computation was not mandatory. Had there been good reason to upgrade its priority, it could readily have been done earlier. The budget stringency that brought this
deferral (along with many others) thus was false economy par excellence, for the program did not halt during that year of launch delay. It kept writing checks for its contractors and employees. The missing tile – strength analysis thus ramified in its consequences, contributing substantially to a cost overrun in the Shuttle program.[626]
During 1979, NASA gave the same intense level of attention to the tiles’ mechanical problems that it had previously reserved for their thermal development. The effort nevertheless continued to follow the pattern of three steps forward and two steps back, and, for a while, more tiles were removed than were put on in a given week. Even so, by the fall of 1980, the end was in sight.[627]
During the spring of 1979, before the main tile problems had come to light, the schedule had called for the complete assembly of Columbia, with its external tank and solid boosters, to take place on November 24, 1979. Exactly 1 year later, a tow vehicle pulled Columbia into the Vehicle Assembly Building as a large crowd watched and cheered. Within 2 days, Columbia was mounted to its tank, forming a live Shuttle in flight configuration. Kenneth Kleinknecht, an X-series and space flight veteran and now Shuttle manager at NASA Johnson, put it succinctly: "The vehicle is ready to launch.”[628]