Although it was an integral part of X-15-3, the Minneapolis-Honeywell MH-96 adaptive flight – control system was also an experiment and hence part of the research program. In 1956, researchers performing in-house studies at the Flight Control Laboratory of the Aeronautical Systems Division (ASD) at Wright-Patterson AFB determined that it was feasible to design a selfadaptive flight-control system. As the name implies, such a system automatically adapts itself to provide essentially constant damping in flight conditions of varying control-system effectiveness. In other words, a given movement of the control stick would always result in the same airplane response, regardless of how far the control surfaces had to move to accomplish the maneuver. At the time, most aircraft still had simple mechanical linkages to the flight controls, with manually set trim tabs. The new supersonic fighters had more sophisticated system that adjusted their gains as a function of measured and computed air data. However, these functions required extensive flight-testing to perfect, and generally resulted in complex and unreliable systems. Researchers expected that future vehicles would be operating in flight regimes where air data might not be available, and decided to develop a new approach. The Air Force awarded a number of study contracts in 1957 that led to flight-testing of a variety of adaptive concepts on several Lockheed F-94 Starfires by the Massachusetts Institute of Technology (MIT) and the Minneapolis – Honeywell Regulator Company. When government funding ended, Minneapolis-Honeywell continued its effort with a company-funded flight program using a McDonnell F-101A Voodoo. The Air Force subsequently provided limited funding for the F-101 trials, and future astronaut
Virgil Grissom flew some of the evaluation flights.*45*
By 1958 the Flight Control Laboratory was convinced of the potential of self-adaptive techniques; however, the performance of the available aircraft was insufficient to test the concepts, particularly the first expected application in the Boeing X-20 Dyna-Soar orbital glider. The logical choice of test platforms was the X-15 because its flight profile was the closest approximation to the Dyna-Soar that was available. Unfortunately, the X-15 program was already in high gear and the Air Force was reluctant to delay the critical hypersonic testing planned for the three airplanes.*461
Despite the lack of an available test platform, the Flight Control Laboratory continued with its development effort. The Air Force released invitations to bid in late 1958, evaluated proposals during early 1959, and awarded to contract to Minneapolis-Honeywell in June 1959. Although the primary purpose of the program was to test the self-adapting technique in a true aerospace environment, researchers also decided to evaluate several features that were recognized as desirable for any production system. These included dual redundancies for reliability, the integration of aerodynamic and ballistic control systems, rate-command control, and simple outer-loop hold modes for attitude and angle of attack. Within a few months, Honeywell flew the prototype MH-96 in the F-101A at Minneapolis and in the X-15 fixed-base simulator in Inglewood.*47*
The basic system consisted of an adaptive controller that contained the various electronic modules and redundant rate gyro packages (each containing three rate gyros-one for each axis). The system also required an attitude reference (i. e., an inertial platform) and angle-of-attack and angle-of-sideslip information. The electronics modules were programmed with an ideal response rate (the "model") for the aircraft, and the MH-96 adjusted the damper gains automatically until the aircraft responded at the ideal rate. Essentially, the gain changer operated by monitoring the limit-cycle amplitude and adjusting the gain to maintain a constant amplitude. A tendency for the amplitude limit cycle to increase resulted in a gain reduction, whereas loss of the limit cycle initiated a gain increase. Lead compensation largely determined the limit-cycle frequency, which had to be higher than the aircraft’s natural frequency but lower than its structural frequency. On the X-15, that worked out to about four cycles per second.*48*
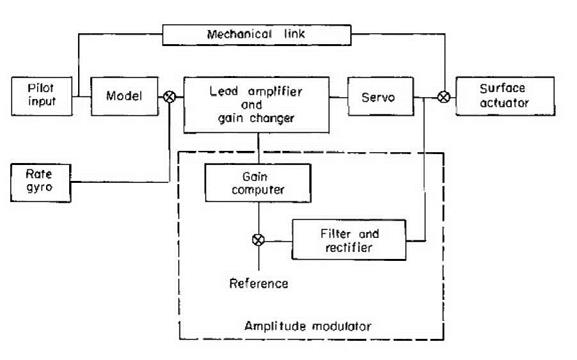
|
The MH-96 adaptive flight control system installed in X-15-3 was an early attempt at a fly-bywire concept, although the linkages were still largely mechanical. Pilot inputs were compared to an ideal model running on a small computer, and the MH-96 commanded the control surfaces to move an appropriate amount based on speed, dynamic pressure, and other variables. The system generally worked well, and the MH-96 was used for all of the program’s high-altitude flights since it provided better redundancy than the standard stability-augmentation system installed in the other two airplanes. (NASA)
Early on, this model presented a problem that was first seen in the X-15 simulator: a quick decrease in gain was necessitated by the rapid buildup of control surface effectiveness during reentry. Delays in the gain reduction, partly caused by the lag in the mechanical control linkages, resulted in temporary oscillations as high as 3 degrees, peak to peak, at the servo. Modifications to the gain computer improved the situation but never eliminated it. However, since the X-20 was going to be a fly-by-wire vehicle, it would not have suffered from this problem. A different issue proved easier to resolve. A control problem existed whenever motions about one axis were coupled to another. To address this, the MH-96 contained cross-control circuitry that commanded a roll input proportional to the yaw rate in order to combat the unfavorably high negative dihedral effect demonstrated by the X-15 during wind-tunnel testing. This was, essentially, the MH-96 flying the beta-dot technique.-49
When a ground test of the XLR99 severely damaged X-15-3, the Air Force agreed to modify the airplane to accommodate the prototype MH-96. The installation of the system into the airplane began in December 1960 and presented something of a challenge. Although it allowed the removal of the original Westinghouse stability augmentation system, the MH-96 required an even greater volume. NASA installed most of the system electronics on the lower instrument-elevator shelf, but this required a "rather extensive revision of the original instrument-recording configuration" since the data recorders normally occupied this area.-501
requirement due to the low probability of a successful reentry from high altitude without damping. The ability to fail safe was equally important since a large transient introduced in a high-dynamic-pressure region would result in the destruction of the vehicle. The MH-96 provided completely redundant damper channels, such that either channel could control the vehicle. The adaptive feature of the circuitry permitted one channel to be lost with little or no loss in system performance, since the remaining gain changer would attempt to provide the additional gain required to match the limit cycle. The gain computers were interlocked, when operative, to prevent overcritical gain following a limit-cycle circuit failure, and to provide the desired limiting effect for hard-over failures.[51]
In the case of a model or variable-gain amplifier failure, conventional monitor circuits would disengage either or both channels when required. Combined with the desire of NASA for increased system flexibility, this led to the addition of parallel fixed-gain channels with fail-safe passive circuitry. Since these channels operated simultaneously with the adaptive channels to avoid the time-lag penalties of switching, they effectively limited the minimum gain for adaptive operation. The fixed-gain circuits had to be sufficiently powerful for satisfactory emergency performance throughout the flight envelope, but below the critical level in the high-dynamic-pressure regions. A successful compromise was elusive, and X-15-3 spent most of its career with some restrictions on its flight envelope.-1521
As installed in X-15-3, the MH-96 provided stability augmentation in the pitch, roll, and yaw axes. The MH-96 controlled both the aero surfaces and the ballistic control system, blending the two as needed to achieve the desired control responses. In addition, autopilot modes provided control-stick steering, pitch and roll attitude hold, heading hold, and angle-of-attack hold. Pilot commands to the system were electrical signals proportional to the displacement of the right side controller or the center stick, and the rudder pedals. Nevertheless, the left-hand controller remained in the cockpit and the pilot could use it if desired to manually control the ballistic control thrusters. Provisions were also incorporated to electrically trim the pitch and roll axes. Trimming presented some problems of its own, mainly because the MH-96 was adapted to use existing X-15 hardware instead of its own newly developed hardware. In order to keep the pitch servos centered, the "trim follow-up" system used a low-rate trim motor to adjust the center point of the pilot’s stick. This not only centered the servos, it also physically moved the stick. If the pilot was performing a precise task while the trim motor was moving the stick, it was easy to get out of phase with the trim motor and it would become saturated, oscillating at low amplitude all by itself at about 0.5 Hz, with no pilot input for several seconds. The pilots found this disconcerting.-1521
The reliability of the adaptive system on the X-15 was excellent, despite the fact that it was not a production system. The mean time between failures of the dampers was 360 hours, and the entire system averaged 200 hours, mainly because some servos were not redundant. The adaptive electronics had a failure time of 100,000 hours. All of these figures compared favorably with the 100 hours demonstrated by the Westinghouse SAS in the other two airplanes.-1541
The MH-96 experienced only two persistent failures during its 65 flights, and each involved only a single axis. It also experienced several other momentary failures on one or more axes; however, in each instance the adaptive mode reengaged following the transient disturbance. Milt Thompson observed that "it appears obvious that black-box technology is capable of providing a high degree of reliability through redundancy and improved electronic hardware." Thompson admitted, however, that the quality of maintenance and the caliber of technical support might have had something to do with the reliability.1551
Although it was not known at the time, 50 years later almost all high-performance aircraft would use some sort of adaptive flight controls. Many of the problems would be eliminated, or at least minimized, by the incorporation of digital fly-by-wire technology, quick-acting hydraulic and electric actuators, and fast digital computers. Nevertheless, given the technology available at the time, the MH-96 was an important first step.