Previous rocket planes, such as the X-1 and X-2, had been able to conduct the majority of their flight research directly over Edwards and the lakebeds immediately surrounding the base. The capabilities of the X-15, however, would need vastly more airspace. The proposed trajectories required an essentially straight flight corridor almost 500 miles long, and the need to acquire real-time data necessitated the installation of radar, telemetry, and communications sites along the entire path. There was also a need for suitable emergency landing areas all along the flight corridor. Fortunately, the high desert was an ideal location for such requirements since many of the ancient lakes had long since vanished, leaving behind dry and hard-packed contingency landing areas.-113
As early as 7 April 1955 Brigadier General Benjamin S. Kelsey wrote to Hugh Dryden (both were members of the Research Airplane Committee) suggesting a cooperative agreement on the construction and operation of a new range to support the X-15 program. A range had been included in the initial Air Force cost estimates, with $1,500,000 budgeted for its construction. At a meeting of the Research Airplane Committee on 17 May 1955, the NACA agreed to cooperate with the WADC and AFFTC in planning the range: the Air Force would build and equip it, and the NACA would operate it after its completion. It was much the same agreement that governed the X-15 itself.114
However, this decision was not favorably received by AFFTC personnel, who felt they were "being relegated to the position of procurement agent" for the XACA. On 15 June, Walt Williams met with the AFFTC commander, Brigadier General J. Stanley Holtoner, to discuss the concept for the new X-15 range. Williams began by updating Holtoner on the status of the X-15 program since the general had not heard any details since the previous October. During this discussion, Holtoner indicated his willingness to cooperate in developing the range and agreed with Williams that the AFFTC should not become actively involved until the XACA was able to discuss "detailed items of hardware" and support. Nevertheless, he felt the AFFTC "should have a somewhat stronger position in the project."113
Despite the apparent lack of enthusiasm for the arrangements within the AFFTC, on 28 July 1955 an amendment to the original X-15 development directive was issued that clearly established the AFFTC’s responsibilities for building the range. However, since neither document discussed which organization would operate the range, the AFFTC renewed its efforts to acquire this responsibility.
A conference at ARDC Headquarters in Baltimore on 15 September 1955 set in place the basic architecture of the range. Technical personnel reviewed the availability of various types of radar and decided that all of the range stations should be similar and include telemetry receivers as well as radar equipment. Although no decision was made regarding the specific radar equipment, the choices were narrowed to the AFMTC Model II used on the Atlantic Missile Range, and the Canoga Mod 3 used by North American at White Sands. On 13 October the HSFS proposed expanding the use of telemetry beyond that used on earlier X-planes. In addition to the normal engine-related information that was traditionally monitored, the HSFS wanted to obtain aircraft information (structural, flight path, temperature, etc.), research data (cosmic ray concentrations, etc.), and pilot physiological effects. This was a stretch for the available technology.-1161
Developing the final specifications for the new range was the subject of a meeting on 16 November 1955. This is when the AFFTC made its move for control, stating that the Air Force would like to operate and maintain the range on the condition that the NACA could also use it for the X-15. The NACA reminded the Air Force that the verbal agreement between Hugh Dryden and General Kelsey had already settled the issue. The NACA representatives also pointed out that the safe operation of the X-15 would depend heavily upon data acquired by the ground stations, and that a division of responsibility would not be desirable. The issue, however, would not go away, and on 2 December 1955 the AFFTC deputy chief of staff for operations at the AFFTC, Lieutenant Colonel Bentley H. Harris, Jr., wrote to the commander of ARDC formally requesting that his center "be assigned the responsibility for operating, as well as developing, the test range." The ARDC reiterated that the NACA would operate the range, but the AFFTC could use it on a noninterference basis.-171
Despite this contentious beginning, in the end the NACA and AFFTC cooperated in planning and using the range. The HSFS instrumentation staff under Gerald M. Truszynski largely determined the requirements based on experience gained during prior research programs. In November 1955, Truszynski informed the Research Airplane Committee that the range should be at least 400 miles long, with three radar stations able to furnish precise data on aircraft position, reentry prediction, geometric altitude, and ground speed. The X-15 required a launch site located near an emergency landing area, intermediate landing sites, intermediate launch sites (for less than full-power/full- duration flights), airfields near the radar sites that could be used for support, and a "reasonably straight course" for the high-speed flight profile.-1181
Besides the technical issues, many other factors determined where the range and its associated ground facilities would be located. Because of the sonic booms, it was not desirable to have the X-15 fly over major metropolitan areas, at least not routinely. Avoiding commercial airline corridors would make flight planning easier, and avoiding mountains would make the pilots happier. Ground stations needed proper "look angles" so that at least one of them could "see" the X-15 at all times. Emergency landing sites had to be spaced so that the X-15 would always be within gliding distance of one of them. The parameters seemed endless.
Truszynski and his staff concluded that the best course lay on a straight line from Wendover,
Utah, to Edwards, with tracking stations near Ely and Beatty, Nevada, and at Edwards. The range would take the X-15 over some of the most beautiful, rugged, and desolate terrain in the Western hemisphere, flying high over Death Valley before swooping down over the Searles basin to a landing on Rogers Dry Lake.-181
All of this led to construction of the High Altitude Continuous Tracking Range, which is generally known simply as the High Range. Officially, the effort was known as Project 1876. The Electronic Engineering Company (EECo) of Los Angeles accomplished the design and construction of the range under an Air Force contract awarded on 9 March 1956. The requirements noted that the "range will consist of a ground area approximately 50 miles wide and 400 miles long wherein a vehicle flying at altitudes up to 500,000 feet can be tracked continuously."-201
Despite the hopelessly optimistic original budget of $1,500,000, the three tracking stations did not come cheap-the more-sophisticated Edwards station cost $4,244,000, and the costs of the other two together were about the same. The Air Force spent another $3.3 million on initial High Range construction, and the NACA would spend a similar amount for improvements over the first few years of operations. An office at Patrick AFB, Florida, managed the procurement of the radar equipment under a modification to an existing contract for the Atlantic Missile Range (later the Eastern Range).-211
The agreement between the NASA and the AFFTC stated that the Air Force would "retain title to the land, buildings, and equipment, except those physically located within NASA facilities." In addition, "control, operation and support of High Range will revert to USAF upon the conclusion of X-15 Flight Research or earlier if the Research Airplane Committee judges that the National Situation so dictates."-221
Although Truszynski and his staff at the HSFS had developed the basic configuration of the High Range, it was up to the EECo-with the advice and consent of the government-to select the actual sites for the tracking stations. Since the HSFS staff had already made rough site selections, the next step was developing a radar coverage map. This map showed considerations such as obstructions on the horizon, the curvature of the Earth, and the range in which a target could be "seen" by radar at specified altitudes. This map narrowed down the area that the EECo needed to investigate in detail. Next came a lot of field work.[23]
Preliminary investigations by AFFTC, NACA, and EECo personnel indicated a possible site called VABM 8002 located 1.5 miles northwest of Ely, Nevada (the number referred to the site’s elevation: 8,002 feet above sea level). However, measurements and photographs from this site taken by EECo personnel indicated that it would not provide the required radar sight lines because of an extremely wide and high blockage angle almost directly downrange from the site. In addition, constructing an access road would have required a "considerable amount" of rock blasting. EECo ruled out using the site.-124
An alternate site in Ely was on Rib Hill. This 8,062-foot-high location was a considerable improvement over VABM 8002 in terms of radar sight lines and the ability to build a road and construct the site itself. The downside was that it was adjacent to the Ruth Copper Pit, and the Kennecott Copper Corporation was already planning to extend the operation into the side of Rib Hill. Even if the hill went untouched, the mining operation would have created too much earth movement for a precision radar installation, so again the EECo ruled out the site.[25]
Fortunately, while investigating the Rib Hill site, EECo personnel ventured to the south ridge of the Rib Hill range. This site was promising because the radar sight lines were excellent. The civil engineering firm of F. W. Millard and Son conducted a detailed land survey, mapping out the best location of the buildings and the access road. The EECo estimated that a 5.65-mile-long, 12- foot-wide road from U. S. Highway 50 to the site would cost approximately $72,400, which included installing culverts and drainage ditches, cutting and filling slopes, clearing and compacting the base, and finishing the gravel road.[26] The road would take advantage of southerly exposures to gain maximum natural snow removal, and arrangements with the White Pine County Road Department and the Nevada Highway Department provided additional mechanical snow removal. It was 10 miles southeast to the town of Ely from the junction of the site access road and Highway 50. The Ely Airport, which was a scheduled stop for several commercial airlines, was five miles east of the town. There were some drawbacks, however. The Kennecott Copper Company offered to supply electricity for a nominal cost, but an evaluation of the mining company’s generators showed that the current could fluctuate 10%, which was unacceptable for the sensitive electronic equipment at the site. EECo estimated that voltage regulators and power lines would cost more than procuring primary and backup generators and generating the required power on-site. In addition, there was no water available at the site, so tank trailers would have to haul water from Ely and store it in a tank at the site.-127
The site at Beatty was somewhat easier to locate. Preliminary investigations by the AFFTC and NACA resulted in the selection of a location approximately six miles northwest of Springdale, Nevada. Further investigation by EECo personnel substantiated this selection. The site was at an elevation of 4,900 feet, approximately three miles west of U. S. Highway 95. The radar sight lines were excellent, and the civil engineering firm of F. W. Millard and Son prepared a detailed survey of the area. Only 1.75 miles of new gravel road would be required to connect the site to Highway 95 at the cost of $30,500, including the installation of culverts and ditches. The site was 20 miles by road from Beatty, and an additional five miles to the Beatty airfield. No commercial power or water was available at the site, so the EECo again installed diesel generators. Water (at no cost,
initially) from the Beatty city water supply was trucked to the site.-1281
RANGE FUNCTIONAL DIAGRAM
r^n
RADAR,
TELEMETER.
VOICE
MICROWAVE AND TELEPHONE LWt INTERCONNECTING CIRCUITS
TRANSMITTED BETWEEN STATIONS
TELEMETERING DATA

TIMING
TELEMETERING DATA
The High Range consisted of three stations: one at Beatty, Nevada, one at Ely, Nevada, and the main station at the High-Speed Flight Station at Edwards. All three sites were interconnected by a sophisticated (for 1955) communications network. Each of the Nevada sites had a "local plot" that could track the course of the X-15 if needed. The general concept of the High Range formed the basis of the later manned spaceflight control networks, not surprising since the same man – Gerald Truszynski – was responsible for the High Range and the initial Mercury network. (U. S. Air Force)
The third site, an extension added to the back of the third floor of building 4800 at the HSFS, was the easiest to select. The construction would extend the building toward the airfield ramp from the existing "Flight Control" room using the exterior doorway as the entrance to the new addition. Initial estimates indicated that 1,200 square feet would be adequate for the intended purpose, but further investigation showed that structural constraints required the addition of at least 1,500 square feet. The additional 300 square feet was necessary to take advantage of the existing second-floor columns for greater support of the third-floor addition. After reviewing the plans, the Air Force and NACA requested that EECo further enlarge the addition to 2,500 square feet, which was the maximum the building could accommodate. The addition contained four rooms of roughly equal size: a monitor room with plotting boards, a radar room, a telemetry and communications room, and a utility/work area. No plumbing was required in the addition since the main building housed adequate restroom facilities and photographic dark rooms.-1291
competitive bid would perform the actual construction after the Air Force secured the land for the two remote sites. For unexplained reasons, the acquisition was not as straightforward as expected. For instance, the original schedule showed completion of the access road to the Ely site by 15 December 1956, but the Air Force ran into unexpected difficulties in withdrawing the site from the public domain, which delayed construction. In the end, it was October 1957 before the road was completed.-1301
At both remote sites, a 100-by-100-foot area was graded and hard-surfaced with asphalt paving and a sealant coat. This graded area was large enough to accommodate the radar shelter, vehicle parking area, and such items as the diesel generator, fuel tanks, etc. Because of the remote locations, officials decided to station permanently a Dodge Power Wagon four-wheel-drive truck at each site to provide transportation to the airfield. These trucks had sufficient towing capability to haul the water trailers, and the four-wheel drive allowed access to the site during inclement weather.-1311
Interestingly, the way the Air Force had written the High Range contracts, EECo was responsible for constructing 800 square feet of each shelter to house the telemetry equipment and "housekeeping" rooms, but the Reeves Instrument Company was responsible for constructing another 800 square feet at each shelter to house the radar equipment. Smartly, in order to avoid too much duplication of effort and to ensure a uniform appearance, the companies decided that one or the other should build the entire shelter. Since Reeves was not interested in facility construction, the honor fell to EECo. This was probably not the optimum solution, however, since Reeves retained the responsibility to construct the radar pedestal itself (which was an integral part of the building structure) because the exact position of the radar antenna was important to the final operation of the radar, and both contractors believed that the radar contractor should build the pedestal.-1321
EECo developed a generic 1,760-square-foot floor plan for the remote sites, although each would diverge somewhat from the ideal due to site-specific considerations. In essence, each building consisted of four large rooms: a radar room, a telemetry room, a room for data transmitting and receiving equipment, and a utility/work area. The building also included a smaller telephone- equipment room and dark room, and even smaller restrooms and closets. Oddly, the telephone room could only be accessed from outside the building. EECo calculated that each site would use approximately 155 gallons of water per day (5 gallons for personnel use, 50 for the dark room, and 100 gallons for the flush-type toilet). A 1,000-gallon tank meant that each site would need weekly water deliveries if it was manned continuously. Extreme weather conditions at Ely dictated that the water be stored inside the shelter to keep it from freezing. The shelters consisted of a metal exterior over an insulated framework and drywall interior, with a wooden false floor installed above a concrete slab to provide a location to run wires and cables.1331
The Ely, Beatty, and Edwards tracking stations had radar and telemetry tracking with oscillograph recording, magnetic-tape data collection, and console-monitoring services. Especially early in the flight program, a backup "communicator" was located at each station in case the communication links went down. Each ground station overlapped the next, and communications lines allowed voice communication, timing signals, and radar data to be available to all. Each station recorded all acquired data on tape and film, and strip charts and plotting boards displayed some of the data locally for the backup communicator.1341
Instrument Corporation modified the three Model II radars (generally called Mod II) and the Air Force supplied them to the EECo as government-furnished equipment. The radars had two selectable range settings: 768,000 yards (436 miles) and 384,000 yards (218 miles). The normal method for acquiring the initial target was to use a remote optical tracker. The antenna pedestal also had provisions for mounting an 80-inch boresight camera. Using a unique (for the period) range-phasing system, two or more Mod 2 radars could simultaneously track the same target without mutual interference.-1351
The radar used a 10-foot parabolic dish that transmitted a 2.5-degree wide beam. Peak power was 350 kilowatts with a pulse width of 0.8 microsecond and a selectable pulse-repetition frequency between 205 and 1,707 pulses per second. The maximum slewing rates were approximately 5 degrees per second in azimuth and 2.5 degrees per second in elevation. These were considered adequate for the X-15, although these limitations were considerations during the selection of launch and contingency landing lakes.-351
Precision azimuth and elevation information was obtained from two optical encoders, and range data came from one electromechanical encoder attached directly to the radar. The optical encoders were 16-digit analog-to-digital converters produced by the Baldwin Piano Company that used coded glass disks to produce a reflected binary (Gray)-371 code. The output of these units was a 16-digit parallel code produced by an internally synchronized flashlamp actuated 10 times per second by the master timing signal. This was the primary precision tracking information obtained from the radar system, and an Ampex FR-114 magnetic tape recorder recorded it in digital format. In addition, a data camera photographed the selsyn dial indications of azimuth, elevation, and range for coarse trajectory information.381
The AFFTC Project Datum system at Edwards provided automated processing for the radar and telemetry data recorded on the magnetic tapes. This was a general-purpose data-reduction computer system developed by the Air Force to accept a variety of input data tapes and generate output tapes compatible with the IBM 704 computers used for data processing. The IBM computer, in turn, provided data on factors such as the geometric altitude, plan position, trajectory position, and velocity. Project Datum was a post-test analysis tool, not a real-time system. Another IBM 704 computer was located at the FRC for processing the oscillograph data from the X-15. Operators transferred the raw data on the oscillograph and photorecorders to IBM punched cards by using manually operated film recorders, and the punched cards generated magnetic tapes.-391
Each of the three tracking sites had a "local" Electronic Associates Model 205J plotting board that showed the position of the X-15 as reported by its local radar, and the station at Edwards had a "master" board that correlated all of the results and plotted the vehicle along the entire trajectory. The local boards at each site could alternately display parallax-corrected data from another station. It is interesting to note that the technology of the day did not allow the parallax from the Ely station to be corrected digitally at Edwards because the results would cause the data receiver register to overflow (i. e., the resulting number would be too large for the available space). Since it was necessary to correct the parallax before displaying the data on the master plotting board, engineers devised a method to alter the analog voltage signals at the input to the polar-to – Cartesian coordinate converter. It was an innovative solution to a technological limitation. The coordinate converter itself was an Electronics Associates Model 484A computer.-401
The X-15 made extensive use (for that time) of telemetry data from the vehicle to the ground. As originally installed, the telemetry was a standard pulse duration modulation (PDM) system capable of receiving up to 90 channels of information in the FM frequency band. A servo-driven helical antenna was located at each range station to receive telemetry data. The antenna was slaved to
the radar to track the vehicle, although it could also be positioned manually using a hand crank. Later in the program, NASA installed auto-tracking telemetry antennas at each site. Ampex FR – 114 magnetic tape machines recorded 40 analog real-time outputs from an Applied Science Corporation Series M telemetry decommutator. Immediately after each flight, the receiving station processed the recorded information onto strip chart recorders. At the very end of the flight program, X-15-3 received a modern pulse-code modulation (PCM) telemetry system, and NASA modified the Ely and FRC sites to process the data (NASA had decommissioned Beatty by that time).[41]
Engineers and researchers on the ground needed to look at some of the telemetry data in real time to assist the X-15 pilot if necessary. They could look at this information in various forms on the data monitor consoles located at all three stations, although Edwards generally conducted the critical analyses. All parameters were presented in the form of vertical bar graphs on two center – mounted oscilloscopes, which allowed rapid assessment of a group of parameters to determine whether the operation was within predetermined limits. Of the total parameters transmitted, researchers could look at any 40 at one time, and the strip charts could display an additional 12 channels.-421
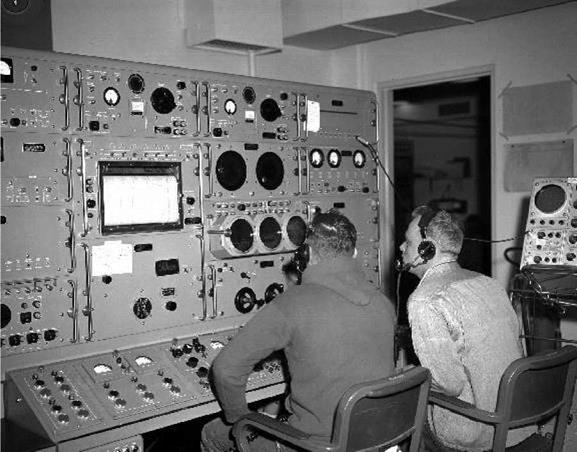
|
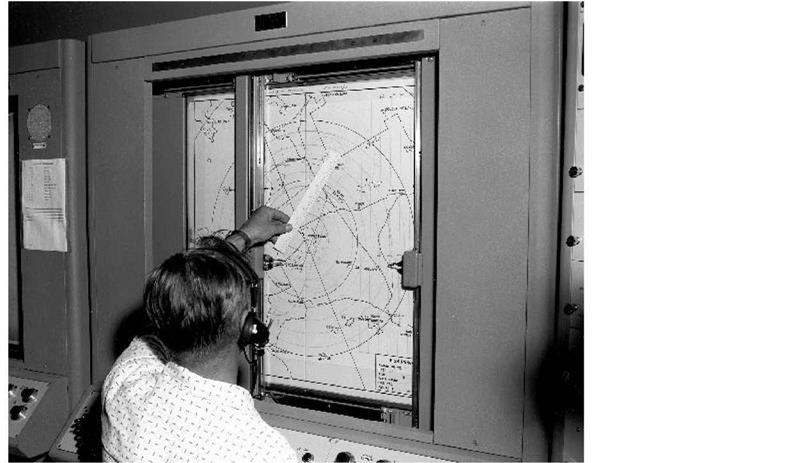
|
When thinking about radar operators, generally a large "radarscope" comes to mind. However, that was not the case during the 1950s, and the output from a radar was generally a small set of oscilliscopes as shown here on the Mod II unit. (It takes a computer to convert raw radar data into a plan-view for display on a radarscope, and such computers largely did not exist during the late 1950s.) For the most part, on the High Range the radar data was processed and displayed on a set of large paper charts that traced the flight progress on a pre-printed map. The position was plotted using one color of ink for position and another for altitude. (NASA)
Standard military ground-to-air AN/GRC-27 UHF equipment provided voice communications with the X-15. Originally, the Air Force indicated that it would provide the radios as government – furnished equipment; however, the long lead times caused the AFFTC to ask EECo to bid on supplying them separately. EECo found a Collins unit with 1,750 channels that it could acquire within nine months. The radio was fully compatible with the AN/ARC-34 UHF transmitter-receiver set that North American would install in the X-15.[43]
To ensure positive contact between any of the tracking sites and the X-15 regardless of its location over the High Range, EECo installed a network communications system. Each range station contained two UHF transmitters and receivers (one of each was a spare) and a specially designed communication amplifier and switching unit. When an operator keyed a transmitter at any location, all three stations transmitted the same information simultaneously. The receivers at all three stations fed their outputs onto a telephone line and, regardless of which station received the information, all stations could hear the transmission. The EECo also installed dedicated station-to-station communications links.[44]
simultaneously, the airborne receiver experienced an "audible beat or tone" interference. The solution to this heterodyne interference problem was to offset each transmitter frequency by a small amount without drifting outside the frequency bandwidth of the receiver. Experimentation led the team to adopt offsets of 0.005-0.010% of the operating frequency as nearly ideal. It was also determined that each transmitter should be offset by an unequal amount to avoid creating a noticeable "beat" in the audio. In the end, technicians tuned the Edwards transmitter 22 kilocycles below the center frequency, while the Ely site transmitted at 14 kilocycles above the center frequency. Beatty, being in the middle, used the center frequency for its transmitter.*451
Since a microphone at any one of the stations modulated all three transmitters simultaneously, the signal arrived at the aircraft at slightly different times because of differing distances from the station to the aircraft. In addition, signals originating on the aircraft took slightly different times to reach each of the ground stations. Consequently, some slightly different delays affected each signal. Given that such signals travel at the speed of light (186,000 miles per second), the time difference for an actual transmission was a maximum of approximately 4 milliseconds. A slightly longer delay was encountered in sending the keying signals between stations, resulting in a total delay of about 12 milliseconds between the two outermost sites (Edwards and Ely).*461
It was found, however, that the time delay was not totally undesirable. The human voice contains a multitude of continuously varying harmonic frequencies. The time delay canceled out a small number of these frequencies since they were 180 degrees out of phase with each other. The only effect this had was to introduce a slight flutter in the reproduced sound that did not seriously degrade speech intelligibility. The second effect the time delay brought was a slight echo effect. Due to the acuity of the human ear, there must be a spacing of approximately 30 milliseconds between signals for the ear to detect that an echo is present. Researchers discovered that a small echo effect actually increases the intelligibility of a voice because of the slight lengthening of word syllables. Analysis indicated that the maximum predicted 12-millisecond time delay would not be sufficient to cause undesirable effects, so the X-15 program elected to ignore the issue.*471
In the course of determining solutions to the various communications challenges, EECo discovered that it was not the first to confront these issues. Commercial airlines had been using similar systems (operating in VHF instead of UHF) for approximately five years after they had installed communications networks under their frequently traveled routes to allow aircraft to be in constant touch with their home offices. Each of these networks was composed of several transmitter – receiver sets that contained between two and six stations tied together by a transmission link. Several groups made up a complete network.*481
United Airlines had designed a similar communications system and contracted its operation to the Aeronautical Radio Company to make it available for other airlines. As Aeronautical Radio expanded and upgraded the original network, it contracted the work to Bell Telephone. Aeronautical Radio leased the system from Bell, and in turn leased the services to the airlines. Collins Radio worked with the service providers and airlines to create a series of radios specifically tailored to operate in the multiple-transmitter environment. Aeronautical Radio, Bell Telephone, Collins Radio, and United Airlines all provided information and assistance to EECo at no charge.*491
In order to evaluate a working communications system of this type before committing to the use of one on the High Range, EECo arranged for a demonstration using one of the airline VHF networks that ran in a line between Oceanside near San Diego to San Francisco, California. The NACA flew a Boeing B-47 Stratojet from Los Angeles to San Francisco at an altitude of 15,000 feet, returning to Los Angeles at 40,000 feet. The pilot made contact with the ground at 10- minute intervals while Air Force, NACA, and EECo representatives located at the Los Angeles
International Airport monitored the two-way communications.1501
The network spanned a distance of 400 miles, but used six stations (instead of the three planned for the High Range) to provide communications down to an altitude of 1,000 feet. Coverage for the High Range was concentrated above 7,000 feet, and one of the goals of the evaluation was to determine how the concept worked at high altitudes. On the return flight at 40,000 feet, it was likely that the B-47 received signals from all six ground stations, and that all six ground-stations received signals from the aircraft. Thus, potential interference was even greater than it would be with the three-station network planned for the High Range. The only effect noted during the evaluation was a flutter or warble at certain locations in the flight path. Researchers played tapes recorded during the flight for numerous pilots and ground personnel at Edwards, and nobody voiced any serious objections. This validated the concept for the High Range, and the EECo began procurement of the various radios, switching units, and other components.1511
The three High Range stations could share radar and telemetry data to automatically direct the next radar in line to the target, and to plot radar data from a remote station on a local plotting board if desired. It was necessary to convert the data from each station into the correct relative position using a set of fixed translation equations, which is one reason why the exact position of each radar antenna had to be precisely determined during construction.1521
The High Range stations were positioned on top of mountains to provide the best look angles for the radar and telemetry receivers. The Beatty, Nevada station was closed when the X-15 program ended and nothing remains at the site except for the concrete slabs where the buildings once stood. (NASA)
There were three likely ways to transmit data between the three sites: a leased wire facility, a scatter propagation system, or microwave transmission.1531 The contract with EECo specifically stated that "the contractor shall investigate the possibility of using a microwave service link for
radar data transmission originating at the Ely site, passing through the Beatty site, and terminating at Edwards Air Force Base." To satisfy this requirement, EECo personnel discussed possible microwave solutions with the Collins Radio, Pacific Telephone & Telegraph Company (PT&T), Philco Corporation, and Raytheon Manufacturing. EECo also discussed the possibility of a scatter propagation system with the same companies, although only Collins provided any meaningful data.[54]
A typical solution to the microwave system provided three main terminals at Ely, Beatty, and Edwards linked together by 10 repeater stations located approximately 30 miles apart. Each location had complete standby power and radio frequency (RF) equipment to ensure reliability. Engineers estimated the propagation delay from Ely to Edwards at 1.8 milliseconds. There were, however, substantial costs to build the system. For instance, each of the repeater sites needed power generators (at least primary, and probably backup). Then there was the cost to build roads to each repeater site; at an average cost of $3,000 per mile for an estimated three miles per site, this came to $90,000. The roads were to be of the same quality as a typical "pole maintenance" road not intended for regular vehicle traffic. The estimated cost of the microwave system was $396,000, and estimated operating expenses were $33,000 per year, not including amortization of the initial installation costs.-55
The propagation scatter system would have involved placing 28-foot-diameter antennas at each of the three sites and bouncing signals off the troposphere. Collins Radio recommended using a UHF system for distances up to 350 miles, and VHF for distances up to 1,200 miles. At the time, the Federal Communications Commission (FCC) had not made any licensing provisions for tropospheric scatter systems since it appeared only the government would be interested in using them. Collins pointed out that each system was custom-made, and the only way to determine whether such a system would work between any two or more locations was to try a Collins Transhorizon System in a van setup between each of the sites. Collins estimated the original system cost at $287,600, not including installation or spare parts. Collins also pointed out that the system was very susceptible to atmospheric disturbances and weather.-155
The leased wire facility would provide telephone lines from Edwards through Los Angeles and Sacramento to Reno, Nevada. From Reno the lines would branch off through Tonopah, Nevada, to the Beatty site, and through Wendover, Utah, to the Ely site. The estimated propagation delay from Edwards to Ely was 10 milliseconds. The standard telephone facilities at Ely and Beatty would be "semi public toll service stations," meaning that they would be on a party-line hookup with the towns of Ely and Beatty. All calls from these telephones would be toll calls (10 cents minimum) with a minimum charge of $5.00 per month. The transmission links were semi-permanently connected lines that would not go through an operator’s patch panel, avoiding the chance of accidental disconnections. Pacific Telephone would provide all of the maintenance.-1571
Ma Bell, being Ma Bell, had charges for everything. The initial construction charge (running the necessary land lines and terminal equipment) would be $55,000, but there was also an "installation charge" of $95 per site to have a technician actually connect the equipment. The total annual operating costs would be $113,790, not including the cost of two standard telephones at Ely and Beatty, which would run an additional $5 each per month. Pacific Telephone also informed the government that if it selected a microwave system, the telephone company would not find it profitable to provide only standard telephone service to the two remote sites-this would be economically practical only if Pacific Telephone provided the entire data transmission contract.-581
system since it did not seem to offer any great cost advantage and represented a largely unknown operational quantity. The microwave system offered low annual operating costs, assuming the system continued to be used for at least six or seven years to amortize the installation costs. Additional channels were readily available with minor expenditures, and engineers considered the link more secure since it was unlikely anybody would attempt to "tap" it. The principal disadvantages of the microwave system were its high initial costs, the possibility that the repeater sites would be inaccessible during bad weather, and that maintenance was the responsibility of the end user (the NACA).[59]
On the other hand, leased telephone facilities offered high reliability and low initial costs, and the telephone company would provide all maintenance. Its principal disadvantages were high annual operating costs and the inability to easily add more channels, particularly high-bandwidth ones.*60*
EECo conducted a cost analysis that included amortization of the initial costs over 5-, 10-, and 20-year periods. The results of this analysis for the "Cost per Channel per Mile per Year" were as follows:*611
|
Initial Cost
|
20-Year
|
10-Year
|
5-Year
|
Microwave (Philco)
|
$396,000
|
$21.90
|
$30.10
|
$46.60
|
Telephone (PT&T)
|
$55,000
|
$48.44
|
$49.57
|
$51.81
|
The total annual operating costs, also based on the three possible amortization options were:*62*
|
20-Year
|
10-Year
|
5-Year
|
Microwave (Philco)
|
$52,825
|
$72,650
|
$112,299
|
Telephone (PT&T)
|
$118,680
|
$121,434
|
$126,947
|
The microwave cost curve dropped sharply in the early years and then leveled off to some degree after 10 years. Additional channels, however, dropped the per-channel cost considerably. This was because the basic investment in a microwave system was in the initial installation; additional channels only required more relatively low-cost multiplex equipment. This reduction, however, only extended until expansion filled the full bandwidth of the microwave system. At this point, the cost would increase greatly because additional microwave equipment would be required. This was not a major concern since the proposed system provided a bandwidth of 100 kilocycles, and the seven required channels only used 21 kilocycles.-*63*
Nevertheless, the Air Force was in the position to make the final decision, and it selected the telephone system. There were four reasons for this choice: 1) the high reliability offered by a utility-maintained system, 2) the high initial cost of the microwave system, 3) the distance and inaccessibility of the microwave repeater sites for maintenance, and 4) the fact that the telephone company maintained all telephone facilities. These reasons were unquestionably valid. However, in reality, the more likely rationale was the simple fact that although the Air Force was responsible for funding the installation of the chosen system, the NACA was responsible for maintaining the system once it was operational. The Air Force, therefore, chose the system that would cost it the least amount of up-front money, with little consideration given to future capabilities or operating costs. By March 1961, even before the Ely station came on line (in April 1961), NASA had opted to install a microwave system between the stations on the High Range. The microwave capability from Beatty was operational in June 1961, with Ely following in January 1962.[64]
A master timing system at Edwards provided a constant time reference for all the tracking stations using three separate timing signals: 1,000 parts per second (pps), 100 pps, and 10 pps. An operator at any station could record timing marks on recordings at all three stations to indicate a significant event for later reference.-65
Early in the program, a pilot staffed each of the High Range sites in addition to the engineers and technicians necessary to run the equipment. The pilot at Beatty used the call sign NASA-2, and the one at Ely used NASA-3. For later flights, pilots often did not staff the remote sites as the communications links between the sites acquired more bandwidth and all involved gained more confidence in the reliability of the systems. Normally, important information from the control room passed to the pilot through the NASA-1 controller, who was usually another X-15 pilot. However, other ground-control personnel had the capability to transmit directly to the pilot in the event of an emergency where there might be insufficient time to relay information through NASA – 1, or, as happened on several occasions, the radio at Edwards did not work properly.-66
Although they were not designed as part of the original control room, researchers added various specialized devices during the flight program. For instance, engineers programmed a small analog computer to take radar-derived altitude, velocity, and vertical velocity measurements and compute the resulting range footprint to assist ground personnel in understanding which contingency landing sites were available at every moment during the flight. A scope-type map display presented the data in the control room. The analog flight simulator generated the data to program this computer. The flight surgeons also gained a dedicated biomedical console.-671
The station at Ely was functionally identical to the one at Beatty, although the physical layout of the two sites differed somewhat due to local environmental conditions. At the end of the X-15 program, the Ely station reverted to the Air Force and continued to play a part in test operations until 1992 when it was finally closed. (NASA)
The High Range underwent a series of modifications over the years. For instance, on 10 March 1967, NASA replaced the Mod II radar at Ely with an improved Reeves Instrument Corporation MPS-19C unit that became operational on 2 May. Wallops Island shipped another MPS-19C during March 1967 for installation at the FRC. At the FRC, the original Mod II had been located on top of building 4800, but engineers deemed this unacceptable because the increased accuracy of the new radar required a firmer base to eliminate vibration and flex. As a result, the new radar was installed a mile or so west, primarily in a new facility with a stiffer base. In addition, in early 1967 NASA upgraded the microwave relay system from Ely to Edwards to handle the higher-bandwidth PCM data from X-15-3. The first successful test (at 144 Kbs) was on 29 March 1967, and the system successfully supported flight 3-58-87 on 26 April.[68]