Dates: 26 July – 7 August, 1971
Crew: Commander Dave Scott CM Pilot Al Worden LM Pilot James Irwin
Command Module: Endeavor Lunar Module: Falcon
Accomplishments: Longest Lunar Stay 66 hours 54 minutes, Landing in the Apennine Mountains
J. L. Chen, How to Find the Apollo Landing Sites,
The Patrick Moore Practical Astronomy Series, DOI 10.1007/978-3-319-06456-7_7, © Springer International Publishing Switzerland 2014
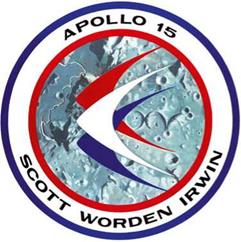
Fig. 7.1 Mission insignia. Courtesy of NASA
|
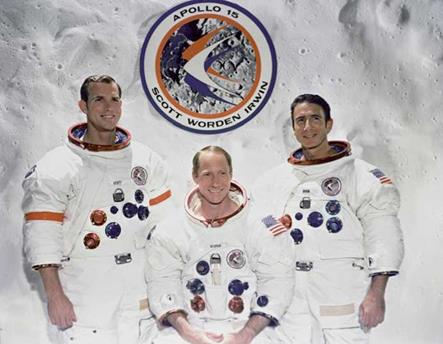
Fig. 7.2 Apollo 15. Courtesy of NASA
|
Apollo 15 was the first of the final three Apollo missions, and the first of the “J” missions. NASA had its funding cut by the Nixon Administration, with Apollo 18 and subsequent missions terminated. In light of the budget cuts, NASA planners placed a greater emphasis on scientific activities for the J missions, and maximized the scientific opportunities of the final three missions.
The J missions were designed to extend the lunar stay for longer periods, conduct lunar exploration over a greater area, and were equipped with a larger array of scientific instruments than on the Apollo 11, 12, and 14 missions. Both the CSM and the LM included major renovations to make room for additional scientific experiments. The installation of a scientific instrument module in one of the service module bays was included for scientific investigations from lunar orbit. The LM received modifications to accommodate a longer stay on the lunar surface and carry a greater payload. The major innovation of the J mission series was the introduction and use of the Lunar Roving Vehicle, or LRV. The landing site chosen for Apollo 15 was at the foot of the Appenine Mountains, and next to Hadley Rille.
As with all Apollo missions, Apollo 15 was not without technical problems that potentially could have scrubbed the mission.
Following the launch, during the initial docking of the CSM with the LM and extraction from the S-IVB third stage, the “SPS Thrust” light on the Entry Monitor System of the control panel came on. The Service Propulsion System (SPS) was the rocket engine of the CSM and the SPS Thrust light was used to indicate that the valves in the engine were open and that the rocket should be firing, something that was not occurring. Just in case, the crew opened the circuit breakers that controlled the valves to prevent them from being opened by a short circuit and initiating the engine to fire.
After some period of troubleshooting, it was determined that there was a short circuit in the “Delta-V Thrust” switch. This switch opened the valves in the SPS. Having discovered that the technical problem was in the switch meant that the engine itself was fine, but new procedures would have to be used when operating the engine to stop accidental ignition.
One of the reasons for the success of the Apollo program was the redundancy of critical systems. In the case of the SPS, there were two independent valve systems for the engine, and with the proper reconfiguration of the valves and switches, any problems could be worked around. Similar redundancies of systems and system reconfigurations enabled successful missions, as seen for instance in the Apollo 12 SCE-to-Aux event. But redundancy has its downside. In a very counter-intuitive concept that is familiar to many logistical engineers, redundancy actually lowers system reliability while increasing the system availability. What? In brief, availability is a measure of the percent of time the equipment is in an operable state, while reliability is a measure of how long the item performs its intended function. A dual redundant system implies two identical systems performing the same function, thus doubling the number of component parts. A failure on one side of the system results in the activation of the redundant system, thus assuring the continued function. With twice the number of parts to assure continued function, the chance of part failure increases and reliability actually decreases. But the redundancy increases the availability of the function.
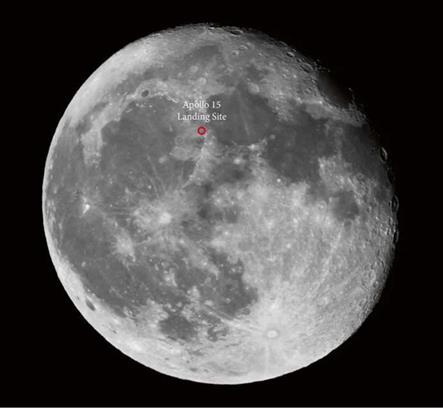
Fig. 7.3 Wide zoom-in of the Apollo 15 landing site. Courtesy of the author
|
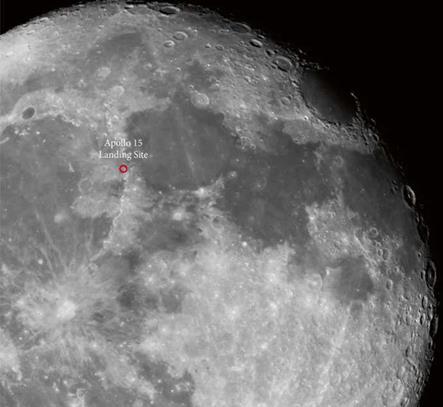
Fig. 7.4 Mid-range zoom-in of Apollo 15 landing site. Courtesy of the author
|
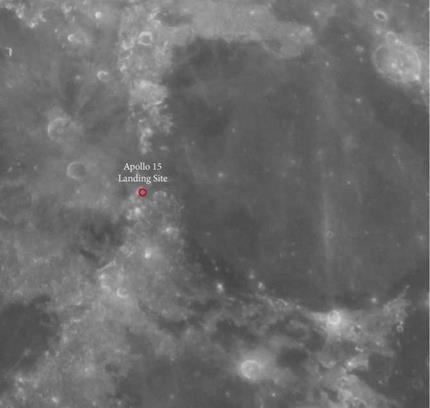
Fig. 7.5 Close-up zoom-in of Apollo 15 landing site. Courtesy of the author
|
The Apollo 15 landing site is a bit of a challenge to spot visually an is shown in Figs. 7.3, 7.4 and 7.5. It is located on the eastern edge of Mare Imbrium, or the Sea of Rains, just south of the “channel” opening to the Sea of Serenity. The landing site is just east of the Julienne Crater and north of the Mons Hadley in the region known as the Mons Hadley Delta. Visually, the telescopic view of this landing site is impressive when compared to the previous three missions because of the tight landing area selected by NASA. Apollo 11, 12, and 14 landed in relatively open terrain, whereas the Apollo 15 site required precision in landing in a restricted area.
Apollo 15, 16, and 17 represented the J missions, which expanded the capabilities for doing science on and near the Moon. NASA planned three 7-hour-long EVAs for Apollo 15, with extensive use of the lunar rover, significantly extending the distance the LM astronauts Dave Scott and Jim Irwin could travel over the lunar surface. Additionally, the restriction on landing near the equator was lifted. A new suite of science experiments was also carried in the service module, giving the CM pilot Al Worden the additional activity of mapping the Moon from orbit.
The landing site chosen for Apollo 15 was on the eastern margin of the Imbrium Basin in the region known as Palus Putredinis. There were two main objectives for this landing site: (1) the rim of the Imbrium Basin could be sampled along the Appenine Mountains, and (2) this site provided an opportunity to explore Hadley Rille, which scientists felt was formed by volcanic processes. Researchers expected that material from Hadley Rille would provide samples from deeper in the lunar crust than was sampled in the Fra Mauro Formation by Apollo 14.
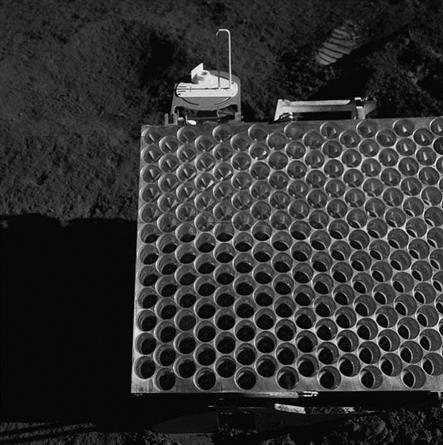
Fig. 7.7 Apollo 15 Lunar Laser Ranging Retroreflector (LRRR). Courtesy of NASA
|
The Lunar Laser Ranging Retroreflector (LRRR) left by NASA at the Apollo 15 site served as a plot point in a popular television show. An episode of the CBS television sitcom “The Big Bang Theory” featured the television show’s characters measuring the distance to the Moon using the Apollo 11 LRRR and a ground based laser. In the series third season, episode 23 called The Lunar Excitation, the “Big Bang Theory” cast is on the roof of their apartment building with a laser, telescopes, electronics, and computers to accomplish the distance measuring experiment.
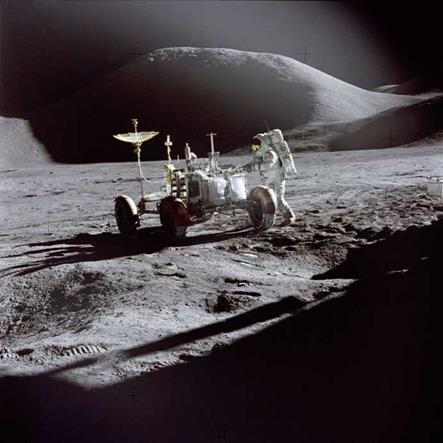
Fig. 7.8 Astronaut James Irwin, LM pilot, works at the Lunar Rover during the first Apollo 15 lunar surface extravehicular activity at the Hadley-Apennine landing site in the shadow of the LM Falcon, and with Mount Hadley in the background. Courtesy of NASA
|
As a precaution, NASA designed the Apollo 15 mission to limit the total radius of the Lunar Rover to 3 miles from the LM. This was done just in case the LRV failed to function (NASA doesn’t build equipment that breaks down! Equipment has a failure or fails to function.), the astronauts would be within walking distance back to the Falcon.
A few years ago, the Lunar Rover was an auction item on eBay as an April Fool’s Day joke, with the auction winner required to pick up the item after winning. The LRV was listed as having very low mileage and driven very little for the past two or three decades. Tires in great shape. A little dusty, but a good car wash would make it look like new. A real collector’s car. No rust and mint condition. No UPS, FedEx, or Parcel Post delivery was available.
The Lunar Rover is still on the Moon, so the auction winner has not made arrangements for pickup yet.
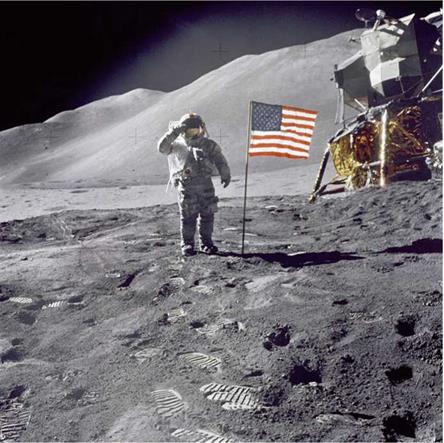
Fig. 7.9 Preparing for the first EVA traverse with the LRV, Apollo 15 LM pilot James Irwin loads-up the LRV with tools and equipment in preparation for the first lunar extravehicular activity at the Hadley-Apennine landing site. The un-deployed Laser Ranging Retro-Reflector (LRRR) lies atop the LM’s Modular Equipment Stowage Assembly (MESA). Courtesy of NASA
|
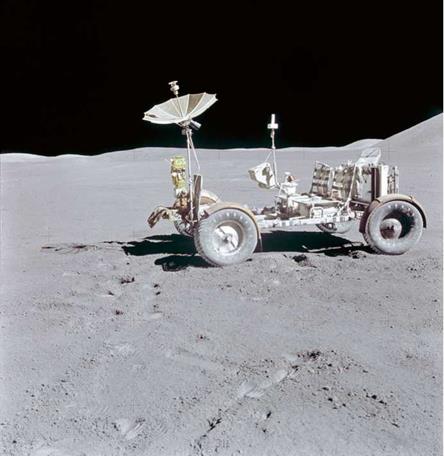
Fig. 7.10 The Lunar Roving Vehicle is still parked at the Hadley-Apennine landing site, waiting pickup by its eBay winner. This view is looking north. The west edge of Mount Hadley is at the upper right of the picture. It rises approximately 14,765 feet above the landing site. Compare this photo with the LRO photo in Fig. 7.6. Courtesy of NASA
|
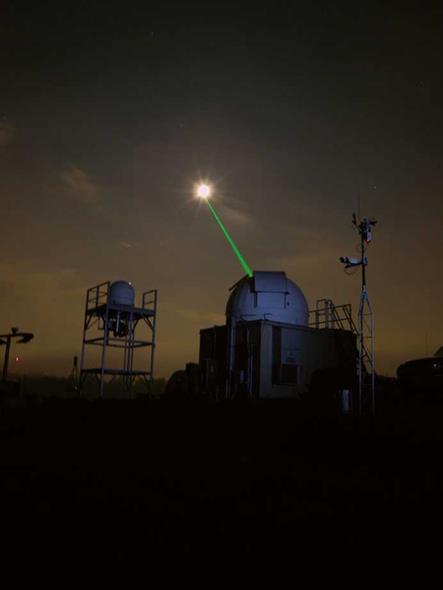
Fig. 7.11 Earthbound laser aimed at the Apollo 15 LRRR, a “Star Wars” dramatic depiction of a ground-based laser measurement of the distance to the Moon using the LRRR left in the Apennine Mountains by Apollo 15. Courtesy of NASA
|
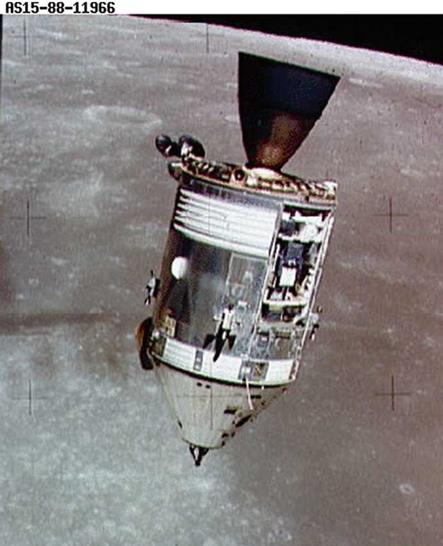
Fig. 7.12 savor with the Scientific Instrument Module open. Courtesy of NASA
|
In addition to their studies on the lunar surface, the Apollo 15 crew performed intensive studies of the Moon from lunar orbit. In addition to photography performed with hand-held cameras in the Command Module, a series of experiments were carried in the Scientific Instrument Module on the Service Module. The same suite of SIM bay instruments was also flown on Apollo 16.
• The metric and panoramic cameras provided systematic photography of the lunar surface. Apollo 15, 16, and 17 carried a set of cameras in the SIM bay.
These cameras were used to obtain high-resolution photographs of the lunar surface, for use both in studying the geology of the surface and for producing detailed topographic maps of the surface.
• The Laser Altimeter measured the heights of lunar surface features. The laser altimeter used a pulse from a laser aimed at the lunar surface. The reflection of the pulse from the surface was then detected with a small telescope. The length of time the pulse took to travel from the spacecraft to the Moon and back translated to the height of the spacecraft above the surface of the Moon. Measurements were made roughly every 30 km across the Moon’s surface. These measurements are sufficiently accurate to distinguish height variations of 10 m between adjacent measurement points. Apollo 15 performed these measurements for a total of 4 1/2 orbits.
• The S-Band Transponder Experiment measured regional variations in the Moon’s gravitational acceleration. This experiment was identical to that carried on Apollo 14.
• The X-ray Fluorescence Spectrometer Experiment and the Gamma ray Spectrometer Experiment measured the composition of the lunar surface. The X-ray Fluorescence Spectrometer detected the ratio of aluminum to silicon and magnesium to silicon in the soil, while the gamma ray spectrometer detected the presence of iron, thorium, and titanium. The X-ray Fluorescence Spectrometer Experiment was limited to the sunlit side of the Moon since it relied on the fluorescence of aluminum, magnesium, and silicon in the presence of X-rays from the Sun. It measured the mare regions, such as the Sea of Serenity and the Sea of Tranquility, and found low abundances of aluminum and high abundances of magnesium. Over the lunar highlands, an opposite pattern of high aluminum abundances and low magnesium abundances were measured. The Gamma ray Spectrometer Experiment had no limitation and could function in both light and dark regions of the Moon. It found high iron abundances over all mare regions and lower abundances elsewhere. Thorium and titanium abundances were also highest over mare regions, but these two elements were not evenly distributed across the maria.
• The Alpha Particle Spectrometer Experiment measured radon emission from the lunar surface. It was flown on both Apollo 15 and 16. The Alpha Particle Spectrometer measured the alpha particles emitted by the surface, specifically by radon-222 and polonium-210 by detecting particles of energies of 4.7-9.1 MeV. When Uranium-238 undergoes radioactive decay, radon-222 and eventually polonium-210 are among the series of lighter isotopes that result. Rn-222 gas has a radioactive half-life of 3.8 days, which is long enough to allow some of it to diffuse through the lunar regolith and enter the lunar atmosphere. A later step in the same decay results in Po-210. Both Rn-222 and Po-210 undergo radioactive decay by releasing alpha particles (the equivalent of a Helium-4 nucleus). The experiment worked by detecting the alpha particles released when these isotopes decayed radioactively. Both Rn-222 and Po-210 were successfully detected in lunar orbit. Apollo 15 detected higher levels of Rn-222 around the Aristarchus
crater area. Higher levels of Po-210 were observed between Sea of Crisis (Mare Crisium) and the crater Van de Graaf on the Moon’s far side.
• The Orbital Mass Spectrometer Experiment (OMSE) measured the composition of the lunar atmosphere. The OMSE was deployed from the SIM on a 7.3-m boom. Most of the gases detected by the OMSE were associated with the Apollo spacecraft itself. However, Neon-20 was detected. Believed to be from external sources, it was about one-third of that expected to be found at the Moon due to capture of gases from the solar wind.
• The Bistatic Radar Experiment measured the scattering of radar waves from the lunar surface. This experiment was identical to that carried on Apollo 14.
• The PFS-1 Subsatellite was a small satellite released into lunar orbit from the SIM bay. The PFS-1 main objectives were to study the plasma, particle, and magnetic field environment of the Moon and map the lunar gravity field. The subsatellite was designed to measure plasma and energetic particle intensities, vector magnetic fields, and facilitate tracking of the satellite velocity to high precision. A basic requirement was that the satellite acquire fields and particle data everywhere on the orbit around the Moon. The Moon’s roughly circular orbit about the Earth at approximately 250,000 miles carried the subsatellite into both interplanetary space and various regions of the Earth’s magnetosphere. The PFS-1 orbited the Moon and remained operational from August, 1971 until January 1973.
• In later years, through a study of many lunar orbiting satellites, scientists came to discover that most low lunar orbits are unstable. Fortunately, the Apollo 15 subsatellite had been placed, unknown to mission planners at the time, very near to one of only four Lunar stable orbital slots known as frozen orbits, where a Lunar satellite may remain indefinitely.
Apollo 15 was the last mission in which a contingency sample was collected. Seven samples taken within 12 m west of the LM Falcon, and were collected to ensure that some lunar material would be returned for study on Earth in the event that an emergency required the rapid, unplanned end to the EVA.
I n addition to their geologic studies, the Apollo 15 crew performed several experiments on the lunar surface. The results of some of these experiments were either radioed to Earth by the crew or returned to Earth for laboratory analysis. The Soil Mechanics Investigation studied the properties of the lunar soil.
Our old friend, the Solar Wind Composition Experiment collected samples of the solar wind for analysis on Earth. Again, the foil used to collect the ions from the solar wind. The sampling foil was collected at the end of EVA-3, stored in the LM for its eventual return to Earth for analysis.
Other experiments were deployed by the crew and then monitored from Earth by radio telemetry after the crew departed.
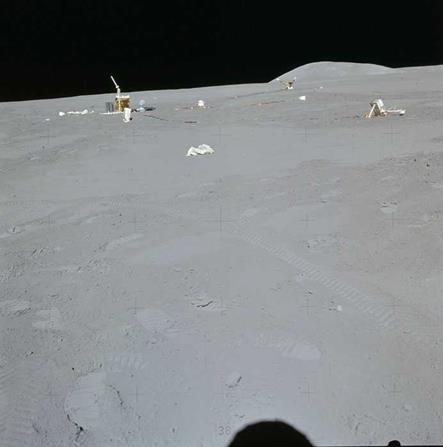
Fig. 7.13 Apollo 15 ALSEP deployed. Courtesy of NASA
|
The Apollo 15 ALSEP included some experiments that were part of previous missions, plus some new investigations were added. Each experiment was connected by a cable to the ALSEP central station, which provides radio communication to Earth and electrical power from a radioisotope thermal generator. Some of these experiments continued to return data until September 1977, when the entire ALSEP network was turned off due to lack of funding for the ground support team.
• Added to the network from Apollo 12 and 14, Apollo 15 ALSEP added another PSE that detected lunar quakes, and provided information about the internal structure of the Moon. This experiment was identical to that carried on Apollo 14.
• The Heat Flow Experiment measured the amount of heat coming out of the Moon. This experiment was identical to that carried on Apollo 14.
• The Lunar Surface Magnetometer measured the strength of the Moon’s magnetic field. On Apollo 12, 15, and 16, the Lunar Surface Magnetometer was included in the ALSEP experiment package.
• The Laser Ranging Retroreflector measured very precisely the distance between the Earth and Moon. This experiment was identical to that carried on Apollo 14.
• The Cold Cathode Gauge measured the abundance of gases in the lunar atmosphere. This experiment was identical to that carried on Apollo 14.
• The Suprathermal Ion Detector Experiment studied the lunar ionosphere. This experiment was identical to that carried on Apollo 14, studying the lunar ionosphere by measuring the various properties of positive ions in the Lunar environment, collecting data on the plasma interaction between solar wind and the Moon, and measuring the electrical potential of the Lunar surface.
• The Solar Wind Spectrometer measured the composition of the solar wind, by continually sampling the solar wind to measure the effects of the Earth’s magnetic field on the constitution of the solar wind. This experiment was identical to that carried on Apollo 12 and 14.
• The Lunar Dust Detector studied the effects of lunar dust on the operation of the experiment package.
And, as in Apollo 14, the Apollo 15 crew also performed the following
experiments:
• The Window meteoroid Experiment studied impacts on the windows of the Apollo 15 Command Module to obtain information about the size distribution of very small micrometeorites. This experiment was identical to that carried on Apollo 14.
• The Light Flashes Experiment studied light flashes seen by the crew that are related to charged particles in space. This experiment was identical to that carried on Apollo 14.
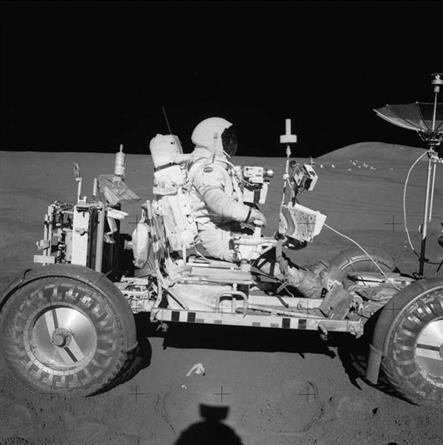
Fig. 7.14 Dave Scott, Commander of Apollo 15, works at the Lunar Roving Vehicle (LRV) during the third lunar surface extravehicular activity (EVA) of the mission at the Hadley – Apennine landing site. Hadley Rille is at the right center of the picture. Hadley Delta, in the background, rises approximately 4,000 m (about 13,124 feet) above the plain. St. George Crater is partially visible at the upper right edge. This view is looking almost due South. Courtesy of NASA
|
After returning to the LM at the end of EVA-3, Dave Scott famously performed a scientific demonstration for the television audience, using a feather and hammer to demonstrate Galileo’s theory that all objects, regardless of mass, in a given gravity field fall at the same rate, in the absence of aerodynamic drag. Scott dropped a hammer and a feather at the same time and the television audience on Earth were able to see both objects land on the lunar surface at the same time. The demonstration worked on the Moon, since there was no drag on the feather due to the negligible lunar atmosphere.
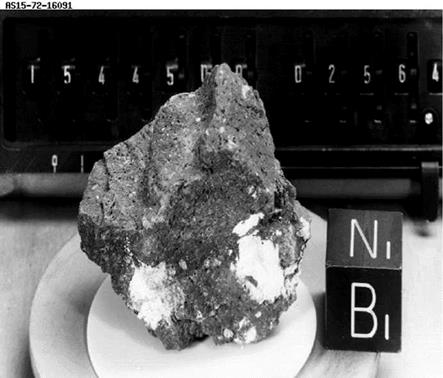
Fig. 7.16 Genesis Rock. Courtesy of NASA
|
During their second lunar EVA, Irwin and Scott retrieved a lunar rock sample that has become known as the Genesis Rock. Apollo 15 sample #15415 became the most famous lunar rock returned by any Apollo mission.
The early excitement of the sample, enhanced by the media dubbing it as the Genesis Rock, was a piece of the Moon’s primordial crust. Chemical analysis of the Genesis Rock has shown it is an anorthosite, composed mostly of anorthite. The dating of the rock was only 4.1 ± 0.1 billion years old, which is younger than the Moon itself. The rock was formed after the Moon’s crust had solidified. Dating of pyroxenes from other anorthosite samples gave a samarium neodymium age of crystallization of 4.46 billion years, with the solar system forming approximately 100 million years earlier.
Although not quite the Genesis Rock as the name implies, the sample is an extremely old rock and was a significant find by the Apollo 15 astronauts. It is currently stored at the Lunar Sample Laboratory Facility at the Johnson Space Center, and is available for study by scientists.
As with all Apollo flights, not all phases of the mission went as planned. Whereas the launch of Apollo 15 was relatively uneventful, one of the parachutes failed to open during the landing. Apollo capsules were designed to land safely with only two parachutes, with the third parachute being redundant in case of failure. With two good parachutes, Apollo 15 landed with a slightly higher velocity of 24 mph, instead of the more gentle 19 mph if all three parachutes had deployed. The post mission analysis of the failure, as reported in the NASA Aeronautics book Coming Home, revealed the parachute lines had been damaged by the fuel from the Reaction Control System, or RCS. The raw fuel from the RCS was vented prior to landing in a depletion firing, and this firing caused a failure in the parachute riser and lines. The failure was regarded as a 1-in-17,000 chance of reoccurring.
Upon landing, Scott, Worden, and Irwin were the first lunar landing crew who were not subjected to quarantine, since the results of the previous missions clearly showed the lifelessness of the lunar environment. The LM Falcon, after returning Scott and Irwin to Endeavor, was jettisoned and impacted the Moon on August 3, 1971 at 26.36° N and 0.25° E. The Saturn S-IVB third stage impacted the Moon on an earlier date of July 29, 1971 at latitude 1.51° S and longitude 11.81° W. Both the Falcon and the S-IVB impacts were recorded by the PSE network which now included the PSE at the newly deployed Apollo 15 ALSEP at Hadley Rille and the Apennine Mountains.