Shaving was optional. Many lunar explorers returned to Earth with two weeks’ growth proudly displayed as they stepped off the helicopter following their recovery. Others chose to shave even though it could be difficult. Mike Collins did a bit of both and returned to Earth with a decent moustache. Although these normally fastidious men tolerated such limitations to their personal hygiene for the duration
of a mission, many began to be irritated by them towards the end and were only too glad to get back to Earth and cleanliness.
The crew of Apollo 10 tried using old-fashioned shaving cream and a razor instead of a mechanical shaver. ‘‘We’re in the process now of commencing scientific experiment Sugar Hotel Alpha Victor Echo [SHAVE],” joked Eugene Cernan, “and it’s going to be conducted like all normal human beings do it.”
Later, during a TV broadcast, Capcom Charlie Duke commented on the pictures coming down of commander Tom Stafford. “Okay, 10. I think we’re looking at Tom’s left shoulder there now, and the Sun coming in his window. Yes. There’s his old grinning face, clean shaven.”
“Roger,” said John Young. “This is a remarkable innovation. After spending a lot of money on mechanical shavers which always manage to leave the whiskers flying around in the atmosphere, somebody finally came out with the idea of using a straight razor and brushless shaving cream. You rub it on, it keeps the whiskers
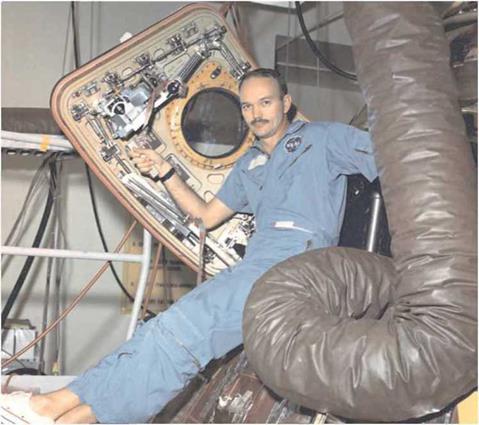
Michael Collins with his Moon moustache at the hatch of Columbia, shortly after being released from quarantine. (NASA)
|
when you shave it off, you put it in a towel and dispose of it, and you end up clean shaven.”
“That’s amazing, 10,” said Duke. “That’s what the space age does for you.” “I’ll tell you, Charlie,” said Cernan, “that’s one of the most refreshing things that’s happened in the last couple of days. That was really great. We were getting where we could barely stand ourselves there for a while.”
However, a continuing problem was dealing with the effects of weightlessness on fluids and the hairs that had been shaved off, as Neil Armstrong explained: “We did shaving on board, and didn’t have a lot of real good luck with that. For some reason or other, we let our whiskers get pretty long before we tried that and found out it was an hour’s job to shave.”
Aldrin elaborated: “It takes a lot more water than you’d think ahead of time, and getting water on your face is not too easy a task. You can get some to accumulate on your fingers in a thin film and then get it on your face, but invariably it’s going to start bubbling and get all over the cockpit in various places.”
“The only difficulty really was conditioning the beard for shaving,” continued Armstrong. “Handling the equipment was no problem and there was no problem with shaving cream getting away from you. It wasn’t that kind of a problem.”
“Well it did use up a fair number of tissues to keep wiping it off,” said Aldrin.
Collins shaved but let a moustache grow. “Now, in 1 g, what you do when you get all through shaving is to bend over the bowl, you take water, wipe it all over your face, and all the bits and pieces of hair go down the sink. But the way we were doing it, when you got through, they were all over your face; then you had to wipe each and every one off. It was sort of hard to get them off. For hours afterwards, they were scratching and itching.”
The Apollo 15 crew decided not to bother with shaving throughout their mission. The increasing length of their beards became obvious just prior to a press conference on the penultimate day of the journey. Karl Henize was Capcom at the time:
“Hey, 15, we’re getting a beautiful picture coming through.”
“Yes,” confirmed David Scott. “Go ahead with your questions.”
“Roger. We’ll admire the beautiful picture for a few minutes here,” complimented Henize.
The crew appeared on camera in a row. Scott was camera-left, A1 Worden in the middle and Jim Irwin on camera-right. Behind them was the lower equipment bay with the optics above Scott’s head and the DSKY between Scott and Worden. All were sporting over 10 days’ growth on their chins.
“Deke just passed out from the shock, incidentally,” joked Henize. They all laughed. Deke Slayton was the crew’s boss in Houston.
“Do we look that scroungy?” asked Irwin.
“No, we look so good,” quipped Scott. “He probably can’t believe it.”
“It’s just because we haven’t shaved in two weeks,” Irwin reminded them.
“Is that a fact?” said the laconic Worden.
Irwin: “Yes.”
By Apollo 16, John Young and his crew were still comparing wet shaving with mechanical shavers.
“I tried the windup,” said Mattingly, “and that worked great until you missed a day. If you miss a day, you’ve had it, because that thing feels like its pulling the whiskers out instead of shaving them off.”
Young had tried wet shaving again, with mixed results. “The Wilkinson worked okay if you’d taken that cream and made a lather out of it.”
“Well, you looked pretty bloody, John, the time you used it,” Duke reminded him.
“You wouldn’t have sold any blades, John,” said Mattingly.
“I really didn’t get too good, did I?” agreed Young. “Pretty bad.”
“Somehow we ought to be able to find a way to let you have a razor that you can open up like any other safely ra/.or and clean off.’’ suggested Mattingly. “That’s the big problem. You get that thing all crudded up and that’s it. There must be some way to do that without producing a free floating hazard.’’
After Apollo 17. Cernan said how important shaving was to the crew. “1 think it’s one of the most clean feelings a guy can get in the spacecraft.’’
Schmitt agreed. "It’s great. I could only shave about a third of the face at a Lime, maybe a fourth, so that’s the way you do it. You put a little bit on and shave that part off and start again. I’ve got a recommendation on the razors. And Gene didn’t have that problem. 1 guess my beard is a little thicker or something, but I couldn’t use a two-bladed razor. I could gel one scrape out of the thing and it was full. There is just no way to clean it out and it just wouldn’t cut anymore. The single-blade razor is the one that evidently has enough room in there. Even though it got plugged up with the shaving cream, it still worked okay."