Upon Van Allen’s return to Iowa City on Friday, 2 May, he, Carl, and I met to plan our work. Additional personnel would be needed, and more student helpers were quickly identified. Arrangements were made with Stuhlinger for ABMA to send engineers to help us on short-term staffing loans. In addition, Huntsville would detail an engineer to our laboratory to help us over a longer term. Importantly, in the process of helping us, those engineers would learn what they needed to know for testing our satellite payloads and integrating them into the launch assemblies at Huntsville.
Our work progressed at breakneck speed. Carl concentrated on developing the new scintillator counters. One was for measuring the average current (representing total integrated energy) over a very wide intensity range, while the other included a pulse
OPENING SPACE RESEARCH
height discriminator to provide information on the particle energy spectrum. One of my early tasks was to develop a faster scaling circuit that would work with GM counters (Anton type 302) having a smaller cross section than the earlier Explorer units by a factor of about 100 times. The scintillation counters and faster GM counters represented the first configuration explicitly designed to follow up on the new radiation belt discovery by making measurements to much higher intensities than had been possible with the earlier Explorers.
Within several days, we had detailed the final detector configuration, and I sent that to Ernst Stuhlinger at Huntsville.15 Within several more days, engineers began arriving from Huntsville. Hermann Wagner was the first—in short order, we were visited by specialists in structures, electronics, environmental testing, and payload checkout, and H. Burke arrived to help us over a longer period.
Relating the details of the many activities that took place during the next weeks would be a book in itself. As already stated, the ABMA at Huntsville carried the primary mission responsibility. I worked extensively with Ernst Stuhlinger, Joseph Boehm, and Charles Lundquist in coordinating many of the programmatic issues. The working-level contacts that I remember with special fondness include (in alphabetical order) Tomas (Tom) A. Barr, H. Burke, George Campbell, Charles Chambers, Harold Donnelly, James Warren Harper, Gerhard Heller, Hans Kampmeier, Samuel (Sam) Stevens (Huntsville’s payload manager), Arthur (Art) Thompson, Willis Underwood, and Hermann Wagner.
I served as SUI’s project manager, overall system designer, coordinator, and equipment and supplies procurer. By mid-project, William (Bill) Whelpley, a young engineering student, had become my right arm, taking responsibility, among other things, for a substantial portion of the field activities. He continued his work in the laboratory for a number of years, becoming a very competent satellite designer in his own right.
Carl McIlwain carried the full responsibility for the new scintillation counters (Figure 13.1), with Ernie Ray working with him from time to time. Carl was also assisted by Dale (Pete) Chinburg, who prepared his numerous drawings. Pete also remained with the Iowa group for a number of years, where he served primarily as a payload coordinator and manager.
Wayne Graves continued his earlier assignment from Collins Radio in Cedar Rapids, providing very skillful help with the electronics design and construction and with GM counter calibration. Charles Cook, Riley Newman, and Chris Richards provided additional laboratory assistance. Drafting support was provided by Mr. Schnerre.
The JPL provided the low-power transmitters, subcarrier oscillators, antenna gaps, and outer shells with their temperature-control coatings. In addition to working with
CHAPTER 1 3
ARGUS AND EXPLORERS IV AND V
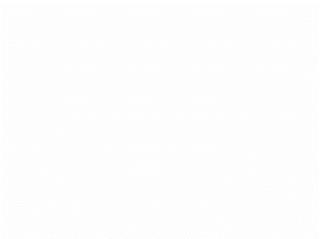
FIGURE 13.1 Carl Mcllwain calibrating the detectors in one ofthe Explorer IV instruments. The detector cluster on the near end ofthe instrument package contains the two GM counters (under the test instrument he is holding). His two scintillation detectors are contained in cylinders beside the circuit board containing his front-end electronics. The black unit at the picture’s lower right is the housing for the X-ray tube being used as the calibration source. (Courtesy of Carl E. McIlwain.)
|
|
director Bill Pickering, chief scientist Eb Rechtin, and senior engineer Henry Richter, I worked at various times on this project with Walt Downhower, Karl W. Linnes, Phil D. Potter, Lee Randolph, Fred Riddle, and Al Wolfe.
The NRL in Washington provided the high-power transmitter. My primary interface there was with my good friend Marty Votaw.
The U. S. Signal Research and Development Laboratory at Fort Monmouth, New Jersey, provided the battery packs through their contract with the Mallory Battery Company. My primary contacts there were Hans Ziegler and A. Legath.
During the instrument development, Pentagon brass and other officials showed up at the physics building from time to time. They returned home incredulous:
Visitors to the University of Iowa during the spring and summer of 1958 were astonished to find that a crucial part of this massive undertaking had been entrusted to two graduate students, and two part-time professors working in a small, crowded basement laboratory of the 1909 physics building. But we knew our business and were in no way intimidated by representatives of huge federal agencies.16
Despite the secrecy, we could build all of the equipment in the open, since the satellite and its instrumentation served officially as an International Geophysical Year (IGY) program to extend our investigation of the natural radiation discovered by Explorers I and III. Only the second mission to study the nuclear blasts was held in strict confidence by a small group of us who were building the instrument. In fact, only Van Allen and McIlwain had access to the full range of details. My knowledge
OPENING SPACE RESEARCH
was limited to a basic understanding of the mission and to details necessary to build the instrumented satellite, test it, and interface it with the launch vehicle.
The Argus Project was helped immeasurably by the assignment of a very high military priority that helped to cut through the red tape and delivery delays. Specifically, it enjoyed a DX-A2 designation, where the DX rating was the highest Department of Defense priority, and A2 designated missile-related contracts. Its high priority enabled us to obtain materials that existed anywhere in the national manufacturing, warehousing, and delivery chain, regardless of who may have ordered them. Our procurement of a complete vibration test system illustrates the benefit of that arrangement. It was a large aggregate of electronic and electromechanical assemblies capable of vibration testing our satellite. It included four racks and a console filled with electronics equipment, plus a vibration exciter on which the equipment to be tested was mounted. Those systems were normally produced at the factory only upon receipt of concrete orders, so that the normal delivery time was substantial. We ordered one in late May, and it was delivered and placed in operation in about six weeks. In order to accomplish that, a system that was being built for another customer was intercepted and delivered to us. That system, shown later in the lower left corner of Figure 15.2c, turned out to be operational too late to help much with the Argus Project, so the Explorer IV and V vibration testing was conducted at Huntsville. Our vibration test system was used extensively over time on later projects.
We had a substantial body of experience with most of the circuits and components used in Explorer IV, as they were similar to those in the earlier Explorers. Carl McIlwain’s new detectors, however, introduced a completely new element. During their environmental testing, failures occurred with a calibration relay and with the Radio Corporation of America (RCA) type 6199 photomultiplier tubes. A summary of the problems on 25 June 1958 indicated that the relay problem had been satisfactorily resolved. Although RCA had made some improvements in the tubes, a complete redesign was not possible. The launches would have to proceed with somewhat less than full confidence in them.
Specifically, after the initial tests, their failure rate was determined to be about 25 percent. The late June report summarized the situation, as it had evolved by that time, by stating that the tube was not designed for the rocket launch environment, that no other suitable tube was available, and that there was no time for complete redesign of the tube’s construction. The RCA was able, however, to make some minor changes, and Carl made an improvement in the exterior mounting arrangement to further isolate the tube from payload vibration. In addition, the vibration test levels were somewhat reduced. Because of the urgency of the mission, the decision was made to launch the payloads with an estimated failure rate still at about 5 percent. Fortunately, Explorers IV and V were launched without instrument failure.
The photomultiplier tube evolving from that design effort served for a number of years as the standard for similar rocket and spacecraft detectors.
On 5 May, Stuhlinger called a planning meeting at Huntsville. Neither Van Allen nor I could go because of the press of our work in Iowa City, but we participated via a telephone conference call. Many issues involving receiving stations, orbital computation, payload weight, and the assignment of responsibilities for providing the various components were worked out. That meeting was followed by another on 10 May, which I did attend. Engineers were there from ABMA, JPL, and the Signal Corps Engineering Laboratory in New Jersey, while NRL participants joined us via telephone. During that meeting, a more detailed delivery schedule was established. It was tied to the requirement that we have our satellite in orbit before the first Argus high-altitude nuclear detonation, then scheduled to occur on 31 July.
During the preparations for the Explorer IV and V launches, we employed light military and chartered civil aircraft to shuttle personnel and equipment between Iowa City, Huntsville, and Washington, D. C. The schedule was too pressing for us to wait for commercial flights. At least 15 of those special flights were made.
On 7 June, Carl McIlwain and I packaged our prototype Explorer IV instrument assembly (Figure 13.2) and carried it via an Army Twin-Beech aircraft to Huntsville, where we test-fitted it into the outer satellite shell and began the process of balancing and aligning it. Two days later, Carl returned to Iowa City, where he prepared and sent
OPENING SPACE RESEARCH
some repair parts to me. I returned to Iowa City with ABMA’s George Campbell and Willis Underwood to make a new circuit board on 14 June, and five days later, I flew again to Huntsville with the result. On 21 June, it was back to Iowa City to work on the flight units.
About three weeks later, on 2 July, Bill Whelpley flew to Huntsville with our first flight unit for an extended stay to help with the testing. The next week saw the rapid shuttling of the flight payloads between Iowa City, Huntsville, and Washington. Flight Payload 1 moved from Huntsville back to Iowa City for transmitter rework, back to Huntsville, thence to NRL in Washington, and finally back to Huntsville. Payload 2 also made a trip to NRL for transmitter modification.
On 9 July, I left again for Huntsville to deliver the third flight unit and to remain for a week for extended testing. The rest of my family drove to Huntsville in our personal car to join me. While in Huntsville, we were the guests of gracious hosts George Campbell and his family.
We had a particularly rough night on 11 July, when we repaired a vacuum problem in flight unit 1 and were finally able to run a satisfactory vacuum test on it. Antenna matching problems with that flight unit were also resolved during the night, and we corrected a problem with the high-power transmitter in flight unit 2 and passed a vibration test with it.
And so it continued during the next days. I finally had a much welcomed short break on 16 July, when I drove our family to Cape Canaveral for the launch. I remember having great fun with Rosalie, Barbara, Sharon, and baby George during the drive with group singing, including many spontaneous variations on “Purple People Eater.”
Bill Whelpley remained at Huntsville to continue the testing on flight units, and Carl McIlwain soon joined me at the Cape. The pace there was just as hectic as it had been at Huntsville. During the next few days, I completed the final inspection and calibration of flight unit 1 and worked with the ABMA, NRL, JPL, and Cape personnel on fitting the payload to the launch vehicle, balance tests, and radio frequency testing and calibration. Other activities, such as working out the countdown procedures and checking ground receiving station readiness, occupied the remaining time leading up to launch.
We soon began concentrating on the final preparations of the most promising of the flight payloads. On 22 July, we completed the last radio frequency interference tests with it mounted on the launching vehicle, as it stood upright in the gantry.
Two incidents remain vivid in my memory that emphasize the informality of that early space flight era. A day or two before the Explorer IV launch, I was called to a meeting with Major General Donald N. Yates, Commander of the Air Force’s Atlantic Missile Range. He had the ultimate responsibility for flight safety. As mentioned earlier, an orbital inclination of 51 degrees had been chosen, compared with 33
CHAPTER 13 • ARGUS AND EXPLORERS IV AND V 373
degrees for Explorers I, II, and III. Those earlier launches involved aiming the rockets slightly south of due east from Florida, where their flight paths were well clear of any substantial landmasses. On the other hand, Explorers IV and V in order to achieve the desired higher inclination, had to be aimed northeast, with their paths passing just off the U. S. East Coast. In fact, the swath that included their intended paths, widened by allowances for reasonable aiming errors, included possible passage over the east coast of Newfoundland.
General Yates wanted to be assured that the flight would be reasonably safe. This was the easiest argument that I ever had to make, as he was just as eager to launch as we were. All I had to do was to mutter a few words about the low population density on its expected course and the improbability of hitting any populated areas if the vehicle should happen to stray, and he quickly said, “Let’s go.”
I especially marvel at the second incident. On 23 August, I performed a careful inspection of the number one flight unit being readied for the Explorer V launch. I found that many of the wires in a bundle of interconnecting wires were nearly broken off where they were soldered to the terminals.
Teflon insulation for electronic circuit wire had just made its appearance. The coating process had still not been perfected for copper, although it was well understood for silver. So the hookup wires that we selected were made up of many fine silver strands. The main problem with that was that the silver strands broke easily when flexed repeatedly. To compensate for that, the manufacturer included a stainless steel strand in the center of the bundle. The stainless steel strand provided strength, while the silver strands provided high electrical conductivity.
Our chosen flight instrument had been reworked so much that the silver strands in many of the interconnecting wires had broken, leaving only the single stainless steel strand intact. That, of course, could not be permitted for the flight. I did the thing that I thought most reasonable under the circumstances: I plugged in my soldering iron and went to work on reconnecting the entire main wiring harness, containing dozens of individual soldered connections.
Under the operating procedures of even those early times, that kind of rework would not have been attempted that late in the launching process. The launch would have been delayed, the repairs made, and a number of the electrical and environmental tests repeated to make sure that the work had been done properly. In this instance, though, time would not permit the standard procedures because of the inflexible Argus schedule. I felt comfortable in undertaking the rework, since I had personally installed the wiring harnesses in the first place.
The Huntsville engineers, I discovered later, were horrified. While I was soldering away, a number of them quietly retreated to a nearby trailer-workshop to discuss the situation. After a lengthy debate, and in consideration of the extremely tight schedule, they decided to let “that crazy Iowa student” proceed with the repairs. I completed
OPENING SPACE RESEARCH
the work in short order, and we proceeded with the remaining preparations as though nothing unusual had happened.
The repair worked perfectly.
With the experience that everyone had gained with the earlier Explorer launches, the countdown procedures had become very well established. We even had a written procedure in case the payload instrument should malfunction during the countdown. It spelled out the precise conditions that would call for either a hold or postponement. Interestingly, my copy was hand annotated “hide,” probably indicating that we did not want to reveal anything to the public other than full confidence in success.
The countdown began early in the morning of 26 July. The launch was completely normal, and Explorer IV was successfully in orbit later that day.
The satellite’s initially computed orbit ranged from 164 miles at perigee to 1381 miles at apogee, with an inclination relative to the Earth’s equator of 50.1 degrees. The initial orbital period was 110.1 minutes. Because of the rather low perigee, it decayed from its orbit and reentered the Earth’s atmosphere a little more than a year later.
With its attached final rocket stage, Explorer IV was, like the earlier Explorers, 80 inches in length and 6 inches in diameter. The total weight placed in orbit was 38.43 pounds, of which 18.26 pounds was the instrument, 7.50 pounds was the shell, and 12.67 pounds was the exhausted final rocket stage. The increased payload weight and higher orbital inclination were made possible by an upgrading by JPL of the high-speed rocket stages. [10]
CHAPTER 13 • ARGUS AND EXPLORERS IV AND V 375
the NRL engineers checked the transmitter and found problems, and a replacement transmitter was flown from Washington and installed. Flight Payload 1 was chosen as the best-performing instrument.
The launch countdown began at 5:30 PM on 23 August, and ignition occurred early the next morning, but with a hugely disappointing result. The final rocket stage failed to ignite, and Explorer V ended up somewhere at the bottom of the Atlantic Ocean.
The day after the Explorer V launch attempt, I detoured to Huntsville for discussions about the IGY Heavy Payload, and two days later, I eagerly rejoined our group in Iowa City.