In addition, the satellite design had to be completed and built. For the 18 days immediately following the Sputnik launch, I was outside the circle of frenzied discussions, negotiations, and design work mentioned above. That situation changed dramatically on Tuesday, 22 October, when I received a telephone call from JPL’s Eberhardt Rechtin. Following up on our discussion in May of matching an abbreviated version of our cosmic ray package to their Microlock system, he briefly outlined their evolving plans and arranged to visit us the following day.
The JPL visitors joined us in Van Allen’s conference room at 4:00 on Wednesday afternoon. Eb happened to be too ill to travel on that day, so Henry Richter came in his place, accompanied by Walter (Walt) J. Downhower. The Iowa attendees at the beginning of the meeting were Ernie Ray, Frank McDonald, Kinsey Anderson, and me (Van Allen was in the South Pacific). Robert (Bob) Parent, from Vern Suomi’s group at the University of Wisconsin, was on campus for a different meeting that morning, and he joined us.
To open the meeting, Henry Richter stated that Eb Rechtin would be visiting the secretary of the Department of Defense two days hence to offer their services, and they wanted to be able to present specific information on scientific instruments to be carried. After that brief introduction, the other Iowa attendees left, and the JPL visitors and I got down to business. They outlined the overall concept of their satellite and the physical environment to be endured by the instruments. We developed a plan for the integration of our instrument that would meet the combined objectives of ABMA, JPL, and the University of Iowa. Their key objective was to place a useful satellite in orbit as quickly as possible. Our primary objective at Iowa remained as before—to conduct the geographically broad survey of cosmic ray intensity that Van Allen had originally proposed.
The discussion quickly led to an understanding that two packages would be launched. The abbreviated package for an initial rush effort would include the basic cosmic ray instrument that we had been discussing at ABMA. That would be followed
OPENING SPACE RESEARCH
by a second launch of our complete cosmic ray package, essentially as it had been designed for Vanguard.
Several other instruments were being considered for inclusion—thermistors to record temperatures at several places in the satellite and an abbreviated version of Vern Suomi’s solar radiation balance instrument from the University of Wisconsin. Following the meeting, however, after Parent had returned to Madison and briefed Suomi, Suomi stated that it would be difficult or impossible to achieve their scientific objectives with an abbreviated package. Since their full instrument with its internal storage could not be accommodated on either flight, further consideration of their experiment was dropped in favor of relatively simple micrometeorite instruments being developed for Vanguard by Edward (Ed) Manring and Maurice Dubin at the Air Force Cambridge Research Center (AFCRC) near Boston.
It was only later that I realized that I had been drawn, unknowingly, into a major institutional power play. As detailed earlier, we had been working since early in 1957 with Ernst Stuhlinger, Josef Boehm, and their staffs at ABMA on their design for a Jupiter C satellite. Although those discussions included JPL engineers, our understanding was that their participation was for the sole purpose of integrating their Microlock system into the ABMA satellite. It remained our full expectation, as well as that of ABMA’s von Braun, Stuhlinger, Boehm, and their staffs, even until early November, that ABMA would have the lead in developing the satellite, including integrating our instrument.
But Pickering and his staff at JPL had their eyes set upon the satellite task. They believed that the payload logically fit within JPL’s mission, as their organization was shifting away from missile development toward high-technology electronics.
So it was the JPL engineers, instead of ABMA staff members, who visited us on 23 October. As my working relationship with JPL progressed over the next few days, I became increasingly troubled that the ABMA engineers were not participating in our discussions. I mentioned that to Pickering during a conversation on 5 November, but he assured me that his staff were working closely with von Braun’s people, and that it was fully appropriate that I continue to work with the JPL staff. I accepted his assurance.
As I learned later, at the time of that conversation, the ABMA people were completely unaware of the JPL aspirations and separate satellite development effort, even though it had been under way for most of a year. They did not learn of that until 9 November 1957, the day after the directive was issued for the Army to proceed with the Jupiter C satellite program.
On that date, General Medaris held a meeting at Huntsville of ABMA, JPL, and Army personnel to clarify the assignment of roles and responsibilities. It was at that time that Pickering made his move. He asked Medaris for a brief private discussion
CHAPTER 8 • GO! JUPITER C, JUNO, AND DEAL I before the meeting, where he convinced the general that the satellite development role should be assigned to JPL.
Pickering went into that premeeting discussion armed with a number of arguments. The JPL work on communications, as exemplified by the Microlock development, was a strong factor. Furthermore, Pickering believed that they had earned the satellite role through their work with ABMA on Orbiter, Jupiter C, the RTV, and, especially, their efforts during the previous two years in helping to keep the Army satellite possibility alive. He must also have pointed out that greater efficiency and probability of success would result from having the entire upper stage endeavor, including the satellite, located at a single institution rather than to have the rocket cluster/satellite/tracking/telemetry interfaces spread between the two organizations.
Pickering undoubtedly revealed during that private premeeting discussion that JPL had been developing their own satellite and that they were much further along in its development than the ABMA staff. Their production of actual prototype hardware, complete except for the scientific instruments, attested to that fact. I suspect that the final arrow in Pickering’s quiver was the revelation that he had already arranged with me at the University of Iowa to shift our instrument from the Vanguard to the JPL satellite effort.
As author Clayton R. Koppes described the premeeting discussion in 1982:
Just prior to the meeting at which the roles would be assigned, Pickering asked Medaris for a few minutes alone. He argued that JPL should build the satellite. The general probably felt the laboratory could handle the electronics work better than Redstone, and he wanted to keep JPL actively in the Army’s orbit. Von Braun’s jaw dropped when Medaris and Pickering walked into the meeting and informed him of the decision, but the collaboration proved fruitful, and there was more than enough work for both teams. The quarter of an hour Pickering spent with Medaris was momentous. If Redstone had built the Explorer I satellite, it would have had a lock on both the missile [booster] and the satellite. JPL would have been relegated to a minor supporting role, chiefly in its [upper stages and] tracking network, from which it would have been highly unlikely to develop into a major space laboratory. Electronics, which had begun shouldering propulsion aside as the laboratory’s dominant activity during the Corporal weaponization, opened a window to space for JPL.8
In 1986, Pickering recalled that particular event in the following terms:
Medaris had a big meeting on 9 November with about twenty people, including Stewart, Froehlich, and myself. At that meeting, he announced that JPL would be involved [with the satellite design]. I think that came as quite a shock to the Germans. . . we could sense the reaction. But we worked very well together, right up to the launching.9
Additional accounts appeared. Author William E. Burrows described the event and its implications in 1998 in the following way:
Having gotten a green light on the launcher, von Braun and his colleagues assumed that they would get to develop the satellite as well. But they were wrong. Pickering, the chief of the Jet Propulsion Laboratory and a tough New Zealander who was educated at Caltech, believed
OPENING SPACE RESEARCH
that given the lab’s involvement with Project Orbiter from the start, it had every right to continue participating in the push to reach space. He therefore convinced Medaris to award the satellite contract to JPL. The general undoubtedly believed that JPL, which by then had solid experience in both electronics and rocketry, could handle the assignment better than his own agency.
Whatever Medaris’ motive, though, his decision would prove to be a momentous one. By allowing JPL to design and build what would turn out to be America’s first spacecraft, he unknowingly played into the hands of a man who had already quietly decided that JPL needed to abandon work on tactical missiles and aim higher. Pickering wanted to build machines that would explore the solar system. As a university [administered] laboratory, he would explain years later, “It was quite clear to us that our future lay on the space program,” not in weapons work, which offered little intellectual challenge.10
I continue to be disturbed by the fact that I had been carried into JPL’s private satellite development effort well before ABMA was aware that JPL was seeking the satellite-building role. I believed that I was still operating within the ABMA-led collaborative satellite-building effort. If I had that period to live over, I would certainly call Stuhlinger to discuss the institutional ramifications. Although that would probably not have changed the outcome, it would have made the process more open.
Thus, in retrospect, during the period immediately following the Sputnik 1 launch, three possibilities existed for us at Iowa. First, we could have remained with the Vanguard program by rejecting the Army proposal altogether. Second, we could have continued with the ABMA-led planning for the satellite. Finally, we could have accepted the JPL offer. From the vantage point of later developments, the JPL alternative that we accepted was by far the best one. Had we remained with Vanguard, we would have had only one launch opportunity, as the small number of launch vehicles being procured for that program was fully subscribed for the vehicle-testing program and the launching of the scientific instruments that were being developed. As events unfolded, being second in the Vanguard experiment queue, our instrument would probably not have had a launch attempt until sometime in 1959. And history shows that the probability of a successful launch would have been small. The overall probability that we would have discovered the radiation belts ahead of the Soviets is essentially zero.
The second alternative would have been equally disappointing. The ABMA satellite plan included only a single satellite design—the highly truncated one. Although that might well have been successfully launched, we would still have had to depend on the Vanguard program for launching the full instrument with its onboard storage. That would very possibly not have succeeded, as speculated above.
The JPL promise of two launches, one quick one with the truncated instrument and a second one with our full instrument, was tremendously attractive. The decision to go with the JPL satellite program was felicitous—ABMA and JPL made good
CHAPTER 8 • GO! JUPITER C, JUNO, AND DEAL I 221
on their promise, including preparing and launching a third launch vehicle after the failed attempt to launch the complete instrument as Explorer II.
Once the decision was made for JPL to take the lead on the satellite, the arrangement worked well. Throughout, Von Braun insisted that the ABMA primary interest was in getting a satellite into orbit, rather than squabbling over roles. On one occasion, when he and some of his senior staff were flying to California in the ABMA Gulfstream for a meeting with the JPL staff, a discussion broke out about a particular question of roles. The staff wanted von Braun to insist on ABMA responsibility for an activity that JPL also wanted. Von Braun would have none of that. His response to them was to restate his general objective and to threaten the staff that, if they wanted to make an issue of the roles question, he would have the plane turn around and fly back to Huntsville. They continued on to Pasadena.11
To return to the chain of events, Richter and Downhower proceeded from our meeting in Iowa City to Washington, where they joined other JPL members on Thursday for a discussion with a bevy of Army and Navy officers at the Pentagon. The membership of that JPL contingent is a little unclear but probably included either Bill Pickering or Eb Rechtin, plus Jack Froehlich, Al Hibbs, Richter, and Downhower, and perhaps Homer Stewart. Rechtin met with Army Secretary Wilber M. Brucker and Defense Department missile coordinator William A. Holaday on Friday to present their Deal plan. On the following Monday, 28 October, Brucker tentatively agreed with the two-satellite Army/JPL approach. Late that afternoon, Rechtin informed me of that agreement, emphasizing that final approval would have to come from the secretary of defense.
It was during that conversation that Rechtin asked if I had the full authority to shift our experiment from Vanguard to the Army program. That question startled me. I had proceeded with full confidence that Van Allen would be enthusiastically in favor of the change if he had been present. After all, he had long viewed the Army’s Redstone rocket as an attractive launch vehicle. The two of us had made a substantial effort to design our Vanguard instrument package so that it could fit in either the Vanguard or the Jupiter C configuration. The satellite instrument that he and I had worked out with Ernst Stuhlinger and his people during the preceding spring and summer was identical in concept with that now being proposed for the first Army launch. And Rechtin had assured me that our full package would be flown on a second launch.
However, when faced directly with that query, I realized that I, a graduate student working on my master’s thesis on what was actually Van Allen’s proposal and project, did not really have the authority to make such a fundamental decision. I had to reply
OPENING SPACE RESEARCH
to Eb that final approval for Iowa’s program change would have to come from Van Allen and offered to find a way to reach him.
The next morning, I contacted Mr. Sedwick in Commander Hearn’s office at the Naval Research Laboratory (NRL). His was the office responsible for coordinating the USS Glacier expedition to the South Pacific. He told me that unclassified messages could be given for transmission to the nearest Naval Communication Center through Western Union, or to the commandant of the nearest Naval District. Classified messages had to be hand carried to either the nearest Naval Communication Center or other military center. I called Eb Rechtin at JPL to convey that information at 11:00.
A little later in the day, Henry Richter called to tell me that the USS Glacier would not be arriving in New Zealand until 10 November and that they were attempting to reach Van Allen on shipboard.
During that discussion, Richter told me that they had received a copy of the letter carrying the army secretary’s approval of the program. With that, and anticipating Van Allen’s favorable response, I proceeded with my planning.
The following day, Wednesday, 30 October, Richard Porter, chairman of the U. S. Technical Panel on the Earth Satellite Program (TPESP), informed me that he had relayed the proposal for flying our cosmic ray instrument, plus the temperaturemeasuring and micrometeorite instruments, to the U. S. National Committee for the International Geophysical Year (IGY), and that the committee had approved. He further stated that he had discussed the change with Homer Newell, overall head of the Vanguard project at NRL, and that he had not raised any objection. Porter told me that a meeting of the TPESP was scheduled for 6 November and that he would relay our requirement for additional University of Iowa funding for the new work.
I was alarmed at one point during that conversation. Porter seemed to be under the impression that there would be only one flight if the first launch attempt succeeded. He heightened my concern by urging that we prepare our complete package for the first flight “if at all possible.” That was not the agreement I had with the JPL people. When I talked with Henry Richter the next morning, I voiced my concern about Porter’s comments, and reemphasized that the complete package with onboard storage was essential for at least one flight. Henry promised to check into the matter, and later reaffirmed the plan for the multiple launches.
Henry also told me during that conversation that JPL had received the first reply from Van Allen but that it was inconclusive. Because of the project’s secret classification, many of the details could not be mentioned in unencrypted messages. To avoid the complications of handling classified messages, JPL had decided to obtain
CHAPTER 8 • GO! JUPITER C, JUNO, AND DEAL I 223
his approval through clear channels. The second terse message that Pickering sent to Van Allen on 30 October read simply:
To Dr. Van Allen. Would you approve transfer of your experiment to us with two copies in spring? Please advise immediately. Signed: Pickering.12
In the absence of information about the recent negotiations and decisions being made in the United States, even with his full knowledge of the earlier Jupiter C planning for our instrument, it was impossible for Van to understand what was being asked. He replied on 31 October:
Dr. W. H. Pickering. Unable to interpret your word transfer due ignorance recent developments. Our apparatus for original vehicle nearly finished. Delighted prepare three sets non-storage type for JPL program. Signed: Van Allen.13
That message implies that Van Allen was still thinking in terms of the earlier work with the ABMA personnel, and that we would still be depending on Vanguard for the full instrument package. Pickering responded with a message that reached Van Allen on 2 November:
Present planning suggests [flying] most of existing equipment but transferring responsibility to us instead of carrying two programs. First experiment would be continuous. Second would be storage type. Suggest you phone me on arrival in New Zealand.14
The next day we all received another huge surprise—the launch of Sputnik 2 by the Soviets! Whereas we had been startled in October by the large weight and size of their Sputnik 1, we were dumbfounded by the size and sophistication of Sputnik 2. Our Vanguard satellites were expected to weigh only 23 pounds. Sputnik 1 had weighed 184 pounds. Sputnik 2 weighed an unbelievable 1121 pounds! Not only that, but it carried a live animal, the dog Laika, in addition to its array of scientific instruments. Even though it was revealed later that no provisions had been made for recovering the dog, and that she died of heat exhaustion after about seven hours in orbit, the feat represented a huge coup by the Soviets.
No longer could anyone assert that Sputnik 1 had been the lucky result of a shortterm crash effort. It was obvious that the Soviets were very serious about the space business. The workers (and Khrushchev, immediately after the Sputnik 1 launch) clearly recognized the impact of what had now become a race to capture the attention of the whole world and were intent on demonstrating and exploiting their scientific and technological prowess. Even more profoundly, inclusion of the dog in Sputnik 2 suggested their strong interest in manned flight.
OPENING SPACE RESEARCH
The pressures for an early American satellite mounted!
When the USS Glacier finally arrived in Port Lyttleton, New Zealand, on 10 November, an additional message from Pickering awaited Van Allen:
Urgently need your approval on proposed change of Ludwig experiment. Porter committee has given their approval to proposal to change experiment to JPL and to modify experiments as agreed upon between Ludwig and JPL.15
Still somewhat uneasy about his lack of solid information, Van Allen addressed a commercial cable to me at Iowa City on 13 November, asking:
Question: Is Pickering plan for our experiment agreeable with you: Please cable answer IGY rep Christchurch.16
Ernie Ray immediately answered the cable on my behalf:
After high-level approval and obvious rearrangement of old program, George left town for extended stay. George quite happy Pickering plans. Hope you say yes.17
With that reassurance, Van Allen immediately wired Pickering:
Approve transfer our experiment accordance JPL plan.18
Thus, on 14 November, only six days after Department of Defense formal approval of the Army’s satellite plan, Van Allen provided his final approval, and we were free to put the plan into effect.
Van told me some time later that his hesitancy in agreeing to the telegram from JPL was to some extent due to his concern that they might be trying to take over the entire experiment, including the scientific analysis.19 Recently, he indicated that he was not as worried as he might have been, because of his belief in Pickering’s innate honesty. That confidence had developed during the many years that the two of them had worked together. Still, Van was much relieved to receive Ernie’s message. [2]
CHAPTER 8 • GO! JUPITER C, JUNO, AND DEAL I 225
Therefore, I accepted the JPL employment offer as the most expeditious way to proceed. During the rest of that frantic week, I had a number of conversations with Henry Richter, and with the JPL Personnel Office, to arrange for my employment. I was hired as an employee of the California Institute of Technology, working at JPL, with a title of research engineer, effective 18 November 1957, and at a starting salary of $700 per month.
Van and I have looked back on that decision on several occasions and agree in retrospect that it would have been much better if I had remained on the University of Iowa payroll. I would have been received at JPL more clearly as a senior colleague, rather than as a junior member of their own technical staff. As a practical matter, that turned out not to be a serious problem, as I could work around any issues by calling Van Allen and letting him work them out with Pickering. However, it did cause me considerable frustration, as the arrangement left me outside some of the project-related activities that would have been helpful.
Returning to the events of late October and early November, we used my pending employment at JPL as the official justification for a rush trip to Pasadena. Although it was carried on the books as a recruiting interview trip, its real purpose was for Deal project planning. In anticipation of Van Allen’s approval of the programmatic shift, I left Iowa City on 1 November, arriving in Pasadena late that evening. The next day, we worked out many of the details of the two payloads and of the ground system needed to track and communicate with them. JPL attendees at that meeting included, at various times, JPL director Pickering, project director Jack Froehlich, Eb Rechtin, and Henry Richter.
The primary result of that meeting was full agreement on the payload and ground station configurations for the two launches.20 The block diagram for Deal I appears here as Figure 8.2. With only minor changes, that configuration was the one eventually flown on Explorer I. A similar diagram was sketched at that time for the more complex Deal II, as described later.
Whereas the Vanguard system had included a single transmitter for tracking and data transmission, two transmitters were planned for both Deal I and Deal II to provide greater redundancy. As an added advantage, the system would employ both the Vanguard Minitrack ground network already being built by NRL, and the new JPL Microlock ground network, thus providing expanded data recovery and additional ground system redundancy.
We envisioned at the 2 November meeting that both the Deal I and II satellites would employ a low-powered transmitter radiating continuously at a frequency of 108.00 MHz, a power level of 0.01 to 0.02 watts, and with a linearly polarized asymmetrical dipole antenna. That system would be included primarily for Microlock tracking and telemetry reception, and secondarily for Minitrack interferometric
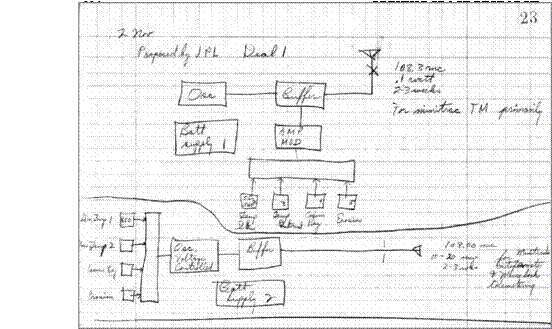
tracking. It would be expected to operate for two to three weeks. By the time Deal I was actually launched in January, the power had been fixed at 0.01 watt, and the design operating lifetime had been increased to “two to three months.”
A relatively high powered second transmitter was to be used on Deal I primarily for transmitting the cosmic ray data to the Minitrack ground network and for interferometric tracking by those stations. It was to radiate continuously at a frequency of 108.03 MHz with a power level of 0.1 watt, for two to three weeks. It would use a circularly polarized X-shaped antenna similar in its mode of operation to the antenna that had been designed for Vanguard. Identical cosmic ray data streams were to be transmitted through both the low – and high-power systems.
The second Deal I experiment, conducted by Ed Manring and Maurice Dubin of the AFCRC, was to study micrometeorites. Micrometeorite density was of considerable scientific interest in its own right. Also, since the space environment was largely unknown, there was a desire to see if very small meteorites were dense and heavy enough to represent a hazard to this and future satellites.
The AFCRC instruments employed two detectors. The first, a microphone placed in spring contact with the satellite’s shell, was tuned to respond to micrometeorite impacts of 0.012 grams per centimeter per second and greater. In more precise terms,
CHAPTER 8 • GO! JUPITER C, JUNO, AND DEAL I 227
the minimum detectable particle mass would be 5.4 x 10-9 grams (assuming a particle velocity of 40 kilometers per second relative to the satellite). The output of that tuned amplifier, scaled by a factor of four, was transmitted via channel 3 of the high-power transmitter.
The second micrometeorite detector was a set of 12 parallel-connected grids of very fine wire. Each grid was wound on a flat bobbin so that it completely covered an area of about one square centimeter. If a micrometeorite of size five microns or larger impinged on one of those grids, its wire would be severed, causing an abrupt change in the resistance of the network. That resistance was telemetered via channel 3 of the low-power system.
The art and science of satellite design was in its infancy, and controlling the temperature of the instruments within the satellite shell represented unknown territory. There was a strong motive for verifying the accuracy of the many calculations and physical provisions for temperature control. To obtain that verification, measurements were made of the temperatures of the aft end of the main cylindrical satellite shell and of the transistor in the high-power transmitter (conveyed over channels 1 and 2 of the high-power system), and of the aft end and tip of the satellite’s front cone (over channels 1 and 2 of the low-power system).
At the 2 November meeting, we also tentatively agreed on plans for JPL to prepare three primary Microlock stations, one near the launch site at Patrick Air Force Base (Cape Canaveral, Florida) and two to be located at then unspecified locations, but “perhaps in Hawaii or the Philippines.” Use would be made of NRL’s full Minitrack stations in South Africa and Australia, plus somewhat simplified Minitrack stations in the United States, South America, New Zealand, Nigeria, and Morocco. That initially envisioned network configuration was eventually considerably modified, as described later.
At the end of that Saturday meeting, we agreed to give urgent priority to procuring certain components that might not be available from supplier’s stocks and might have to be manufactured. Other matters, including arrangements for the University of Iowa to provide the flight tape recorders, security, and financial arrangements, were also addressed.
I spent part of the next morning familiarizing myself with the Pasadena area so that I would be prepared to search for a temporary home when I arrived with my family. That Sunday afternoon, I returned to Iowa City.
The next day, I called Pickering with my proposed budget for the additional Iowa work. Our total budget for developing the satellite instrument as part of the Vanguard Project had been $106,375. I estimated that we would need an additional $39,100 if we were to modify the package at Iowa City. That included an additional $2000 for two
OPENING SPACE RESEARCH
part-time employees for six months, $12,000 for additional equipment and supplies, $8000 for additional travel, and $12,000 for data reduction, which, we realized by then, had not been adequately funded in the original Vanguard budget. I also informed him that, if the work on preparing the instrument were shifted to JPL, the University of Iowa would need only the additional $12,000 for data reduction.
Those funding figures highlight an interesting aspect of the space program at that time. We, on our university campus, and JPL, in Pasadena, operated in entirely different financial realms. During the preceding seven years, the Iowa Physics Department under Van Allen’s leadership had developed a burgeoning space research laboratory from nearly nothing, on a shoestring budget. We were used to scrounging military surplus sources for resistors, capacitors, screws, and other parts, and to operating with a tiny staff of a few faculty members and graduate students and a handful of parttime students. Improvisation was the byword. The total University of Iowa budget of just over $100,000 for the Vanguard instrument (admittedly not including instrument shop expenses and overhead charges) was laughable by later standards, even within a university environment.
On the other hand, JPL was a major military contractor. By that time, it had a staff of over a thousand and was well versed in the business of developing and testing ultrareliable equipment to meet military standards. They were able to commit hundreds of people to the Deal project. A 20 November 1957 Deal organizational chart lists 26 people in senior supervisory and consultative roles, and about 100 others with major named responsibilities, split almost evenly between rocket preparation, satellite instrumentation, and ground stations and operations. Those individuals were backed by legions of technicians and other supporting staff.
This is by no means a criticism of JPL. We would have been terribly hard-pressed at Iowa to prepare our cosmic ray instrument in time to meet the Deal schedule. In addition, the JPL effort provided a much greater assurance of reliable performance for several reasons. The design of all elements of the configuration was worked over meticulously by the highly skilled and experienced JPL engineers, the testing was thorough, and the combined Microlock and Minitrack tracking and telemetry configuration was far more robust than the Vanguard Minitrack system would have been by itself.
In summary, the move of our instrument construction from Iowa City to Pasadena was the only viable arrangement under the circumstances.
On 6 November, immediately upon my return from Pasadena, my direct participation in the Deal project began in earnest with a long working telephone conversation with Henry Richter. He informed me that they were starting work on our Deal I cosmic ray instrument immediately, that Dean Slaughter and John A. Collins would be working
CHAPTER 8 • GO! JUPITER C, JUNO, AND DEAL I 229
with me, and that they were listening in on the line. Those senior engineers in Henry’s New Circuit Elements Group did much of the work in constructing my instruments, testing them, and integrating them into the satellite. They were to become among my closest and most highly regarded associates during my stay at JPL.
Henry’s and my conversation on that day covered some of the preliminary work that they would accomplish before my arrival. As their first priority, they were procuring a supply of the Geiger-Muller (GM) counters. They had already talked to Anton Laboratories, manufacturer of the counters, and the first shipment had been promised for delivery in about a week. His staff were also starting to build a test model of the 700 volt high-voltage supply to power the Geiger counter. Following our discussion, I sent them regulator tubes, transistors, and a transformer so they could breadboard the high-voltage power supply according to the design worked out at the U. S. Army Signal Corps Engineering Laboratories. I immediately called George Hunrath there to coordinate their supply of additional parts kits. Having just made another design revision to improve the voltage regulation, they were nearly ready to ship the kits for the flight instruments.
My time from then until I left Iowa City in mid-November was fully consumed by continuing project coordination and in preparing for my family’s move.
Security was a major concern. Whereas the Vanguard project had been conducted openly, the program to tool up the Army’s Jupiter C satellite launch vehicle was shrouded in secrecy. Recurring delays in the Vanguard launch schedule, the public outcry following the successful USSR Sputnik launches, and the strong element of risk in undertaking the Jupiter launch on such a short schedule made the Army leadership gun-shy, and they chose to operate outside the public spotlight.
That was strongly reinforced on 6 December 1957 following the spectacular and highly publicized launch failure ofVanguard Test Vehicle 3 (TV-3). On that memorable day, with the whole world watching on live television, we were expecting to witness the launch of the first U. S. satellite. Instead, the first stage rocket lost thrust after two seconds, the entire vehicle collapsed into a huge fireball, and the still-bleating satellite spilled onto the ground. The press had a field day!
With that grand public humiliation, the Army became absolutely insistent that details of the Deal project be kept under wraps until a successful launch was achieved. General Medaris at Huntsville strove to make the launch preparations look like just another routine Redstone missile test. Even in highly classified cables between Huntsville and JPL, the launch vehicle was referred to simply as Missile 29. Key personnel whose movements might give away the secret moved under elaborate decoy plans. At the Cape, erection of the booster and the upper stages was particularly sensitive, as that could be observed from the public beaches. Thus, the upper stages were
OPENING SPACE RESEARCH
covered with canvas for their predawn move from the assembly area to the launch pad. The working platforms and shelters on the gantry were kept in place around the upper portion of the assembly throughout the prelaunch preparations to screen it from the beach observers.
Medaris warned, “I cannot overemphasize the importance of these decoy plans and the absolute necessity of covering this launching as a normal test of a Redstone missile, and I desire it well understood that the individual who violates these instructions will be handled severely.”21
Parenthetically, I never saw that directive and was not fully aware of the force of those instructions at the time, understanding simply that we were not to talk about the project outside of our close circle of coworkers. I came close to violating security on one occasion. During a trip from Pasadena to the Cape (it was for either the Explorer II or III launch), our plane was diverted from Orlando to Tampa because of weather. JPL arranged for a car to drive us from Tampa to Cocoa Beach. During that long drive, believing that all in the car were JPL employees, I began to talk about the upcoming launch. I was immediately interrupted by one of the occupants and told to be quiet. Apparently, the driver was not an appropriately cleared JPL employee.
Thus, I had to be discreet in preparing for our move to California. All conversations related to the move had to be cloaked in oblique terminology. I was permitted to include only the head of the Physics Department in any detailed discussions. Ernie Ray was the acting department head in Van Allen’s absence, and we worked closely together on the details. I withdrew from my university classes without being able to give my professors or classmates any cogent reason. Likewise, we withdrew Barbara from her kindergarten class. My Dad, by now an avid satellite program enthusiast and publicist, had been interviewing me frequently on his daily radio programs and had otherwise kept his audience fully informed of our progress. Suddenly, I had to cut off his source of information, and he could mention only that my family was making a sudden move to California.
The other media, especially the local newspapers, had been carrying extensive coverage of our instrument development. They, too, were suddenly cut off from further information. I had to cancel all plans for public appearances. Rosalie and I made hasty arrangements to move out of our house for several months without being able to tell our landlady the reason. Fortunately, our neighbors when we lived in married student housing at Finkbine Park, Gene and Charlotte Boley, needed a house until Gene graduated in February. Thus, they stayed in our house for the initial period of our absence.
The Defense Department made a guarded public announcement on Friday, 8 November, of the president’s decision to launch a satellite with the Army’s vehicle.
CHAPTER 8 • GO! JUPITER C, JUNO, AND DEAL I 231
The formal enabling teletype message reached ABMA the next day. That official go-ahead started the countdown clock. Von Braun had promised that the satellite would be launched within 90 days of approval. Because of scheduling considerations for Cape Canaveral’s downrange facilities, the launch actually had to be planned for 29 January, only 80 days after the approval.
From then on, it was a race against the clock. Work on the instrument repackaging progressed briskly at JPL. I gambled by dispatching some of our household furnishings to Pasadena via moving van on 12 November, two days before receiving Van Allen’s final approval for the program change.