Campaign objectives:
OKB-1 started development work on the Ye-7 lunar orbiter at the same time as the Yc-6 soft lander, but it progressed more slowly. After NPO-Lavochkin took over the robotic program the Soviets became anxious to upstage the American orbiter, which was scheduled for its first launch in mid-1966. They also needed to acquire close up imagery of potential landing sites and information on the environment in lunar orbit for the manned lunar program. An incentive presented itself in early 1966 when the long duration manned Voskhod 3 flight that was to have coincided with the opening of the 23rd Congress of the Communist Party in April (the first for new Communist Party leader Leonid Brezhnev) was canceled and there was a requirement for a new’ space spectacular. The Ye-7 was not ready, but Babakin offered to produce a lunar satellite by replacing the lander of the Ye-6 with a pressurized module carrying a payload of readily available instruments. The first orbiter, the Ye-6S, was cobbled together in less than a month. It is possible that the orbiter module was adapted from an Earth satellite of the Cosmos series.
After a launch failure on March 1, 1966, a backup spacecraft was prepared and successfully dispatched to the Moon on March 31, fortunately for Babakin just in time to satisfy the political objective. By becoming the first lunar orbiter, Luna 10 marked another milestone for the Soviet space program. In a moment of theater, it played a recording of the uInternationale’ to the Party Congress.
The US sent its first orbiter to the Moon a little over 4 months later. This project was successful on the first try, and Lunar Orbiter 1 sent back the first pictures from lunar orbit. With landers and or biters returning high quality data, the US was finally catching up.
Spacecraft launched
First spacecraft:
|
Yc-6S No.204 (Cosmos 111)
|
Mission Type:
|
Lunar Orbiter
|
Country і Builder:
|
USSR NPO-Lavoehkin
|
Launch Vehicle:
|
Molniya-M
|
Launch Date: Time:
|
March 1, 1966 at 11:03:49 UT (Baikonur)
|
Outcome:
|
Failed to leave Earth orbit.
|
Second spacecraft:
|
Luna 10 (Ye-6S No.206)
|
Mission Type:
|
Lunar Orbiter
|
Country j Builder:
|
IJSSR’ NPO – L avoc h к і n
|
Launch Vehicle:
|
Molniya-M
|
Launch Date; Time:
|
March 31, 1966 at 10:47:00 UT (Baikonur)
|
Encounter Date; Thue:
|
April 3, 1966 at 18:44 UT
|
Mission End:
|
May 30, 1966
|
Outcome:
|
Success.
|
Third spacecraft:
|
Luna 11 (Ye-6LF No. lOl)
|
Mission type:
|
Lunar Orbiter
|
Country і Builder:
|
USSR, /NPO-Lavochkin
|
Launch Vehicle:
|
Molniya-M
|
Launch Date: Time:
|
August 24, 1966 at 08:03:00 UT (Baikonur)
|
Encounter Date і Time:
|
August 28, 1966 at 21:49 UT
|
Mission End:
|
October 1, 1966
|
Outcome:
|
Successful orbiter, but no images returned.
|
Fourth spacecraft:
|
Luna 12 (Ye-6LF No. 102)
|
Mission Type:
|
Lunar Orbiter
|
Country! Builder:
|
USSR NPO-Lavochkin
|
Launch Vehicle:
|
Molniya-M
|
Launch Dale ‘: 7 "une:
|
October 22. 1966 at 08:42:00 UT (Baikonur)
|
Encounter Date; lime:
|
October 25. 1966
|
Mission End:
|
January 19, 1967
|
Outcome:
|
Success.
|
Fifth spacecraft:
|
Ye-6LS No. 111 (Cosmos 159)
|
Mission Type:
|
Lunar Orbiter Test Mission
|
Со untry і Builder:
|
USSR NPO-Lavochkin
|
Launch Vehicle:
|
Molniya-M
|
Launch Dale! 7 "une:
|
May 16, 1967 at 21:43:57 UT (Baikonur)
|
Outcome:
|
Earth orbit test mission, lower orbit than desired.
|
Sixth spacecraft:
|
Yc-6LS No. 112
|
Mission Type:
|
Lunar Orbiter
|
Sponsoring Agency:
|
USSR NPO-Lavochkin
|
Launch Vehicle:
|
Molniya-M
|
Launch Date: Time:
|
February 7, 1968 at 10:43:54 UT (Baikonur)
|
Outcome:
|
Stage 3 failure. Did not reach Earth orbit.
|
Seventh spacecraft:
|
Luna 14 (Ye-6LS No.113)
|
Mission Type:
|
Lunar Orbiter
|
Country і Builder:
|
USSR NPO-Lavochkin
|
Launch Vehicle:
|
Molniya-M
|
Launch Date; Time:
|
April 7, 1968 at 10:09:32 UT (Baikonur)
|
Encounter Date/ Tі me:
|
April 10, 1968 at 19:25 UT
|
Mission End:
|
June 24, 1968
|
Outcome:
|
Success.
|
Having achieved lunar orbit ahead of the US and provided the required space spectacular for Moscow, Babakin resumed work on the Ye-7 orbiter whose task was lunar photography. When it was decided to use the Ye-6 cruise stage to make the midcoursc and orbit insertion maneuvers, the orbiter spacecraft became the Yc- 6LF.
Luna 10 had significantly deviated from its predicted path in lunar orbit, and radio tracking had shown the Moon to have an irregular gravity field. As a manned lunar lander would require a precise orbit if it were to land at a preselected point, in
addition to lunar photography the Ye-6LF was given the task of accurately mapping the lunar gravity field.
Two of these spacecraft flew as Luna 11 and 12, and although both achieved lunar orbit an attitude stabilization problem prevented Luna 11 from providing any useful imagery. Even with new tracking data, the navigators could not predict their orbits with the desired accuracy. A second modification to the orbiter module produced the Ye-6LS that was to acquire more precise lunar gravity field data and also test deep space communications for the manned lunar program. After a test mission in Earth orbit and a lunar attempt that was lost to a launch vehicle failure, the third Ye-6LS was successful as Luna 14.
Spacecraft:
These orbiters all used the Ye-6 cruise stage with the lander replaced by an orbiter module. An interesting feature of the orbiters is that they carried no solar panels for recharging batteries, with the result that their operating time was defined by battery life. Lunar orbit insertion was a much smaller burn than the braking maneuver for a landing mission. In the case of the Ye-6S Luna 10, the orbiter module was released on its own in a spin stabilized condition. For the Ye-6LF and Ye-6LS, however, the orbiter modules of Luna 11, 12 and 14 were retained so that they could be stabilized to perform photography. The Ye-6S orbiter module was 1.5 meters long, 0.75 meters in diameter and massed 248.5 kg. It carried two radios transmitting at 18.1 and 922 MHz. Including the cruise stage and a large conical equipment module the Ye-6LF orbiters were 2.7 meters long and 1.5 meters in diameter. The Ye-6LS was similar to the Ye – 6LF but with an improved navigation system to more precisely measure the orbit and apparatus to test a communication system intended for the manned lunar program.
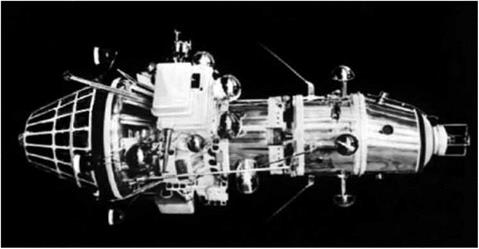
|
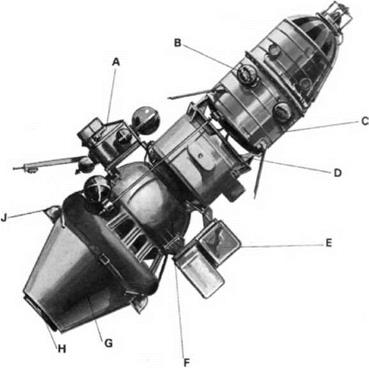
Figure 10.9 Luna 10 Ye-fiS spacecraft (from Robot Explorers): A. Attitude control and radar altimeter; її. Orbiter omni antenna; C. Orbiter module; D. Avionics and communications module; E. Attitude reference sensors; F. Propellant tank; G. Propulsion system; H. Engine nozzle; J. Attitude control jets.
|
|
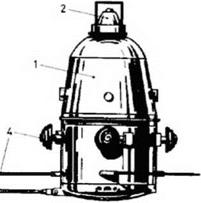
Figure 10.10 Luna 10 orbiter module (from Space Travel Encyclopedia)-. 1. Pressurized instrument module; 2. Radiometer; 3. Magnetometer; 4. Antennas.
|
|
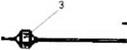
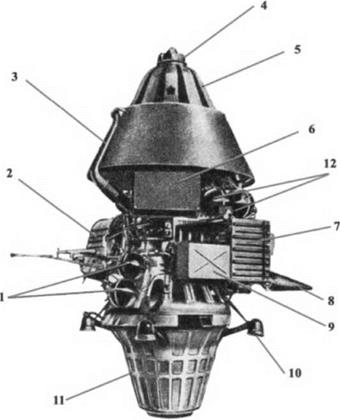
Figure 10.11 Luna 11 and 12 Ye-6LF spacecraft: 1. Helium tanks for attitude control pressurization; 2. Imaging system; 3. Thermal control radiator; 4. Radiometer; 5. Instrument module; 6. Battery; 7. Attitude control sensors; 8. Omnidirectional antenna; 9. Attitude control avionics; 10. Attitude control engines; 11. Main engine; 12. Propellant tanks.
|
|
Luna 10 launch mass: Luna 11 launch mass: Luna 12 launch mass: Luna 14 launch mass:
Payload:
Ye-6S Luna 10:
1. Boom-mounted triaxial fluxgate magnetometer
2. Low energy x-ray spectrometer
3. Gamma-ray spectrometer
4. Gas discharge counters
5. Ion trap solar plasma detectors
6. SL-1 radiometer
7. Micrometeoroid detector
8. Infrared radiometer
9. Gravitational field mapping experiment (using spacecraft tracking)
The Yc-6S flown as Luna 10 had seven instruments developed for the Ye-7, but not the camera. The magnetometer was on a 1.5 meter long boom. Low energy x-ray and gamma-ray spectrometers were to measure the composition of the lunar surface; piezoelectric sensors were to measure micrometeoroid fluxes; an infrared radiometer was to measure thermal radiation from and the temperature of the lunar surface; gas discharge counters were to measure solar and cosmic rays in the lunar environment and soft electrons in a lunar ionosphere; ion traps were to measure electrons and ions in the solar wind and search for a lunar ionosphere; and the SL-1 radiometer was to measure the lunar radiation environment. Another key investigation was to measure the lunar gravity field by radio tracking of the spacecraft. There was no imaging on this first hastily prepared orbiter.
Ye-6LF Luna 11 and 12:
1. Facsimile imaging system
2. Low energy x-ray spectrometer
3. Gamma-ray spectrometer
4. SL-1 radiometer
5. Micrometeoroid detectors
6. Ultraviolet spectrometer
7. Long-wave radio astronomy experiment
8. Gravitational field mapping experiment (using spacecraft tracking)
9. Lunar rover wheel drive technology experiments
The imaging system was similar to the facsimile film camera used by Zond 3 the previous year. At the altitude at which the pictures were to be shot, an image would encompass an area of 25 square kilometers and the 1,100 scan lines would provide a maximum surface resolution of 15 to 20 meters. Two cameras were carried on each mission. The ultraviolet reflectance spectrometer was to measure the structure of the surface. Ко data was ever published on the composition of the lunar surface or of the magnetic field, radiation and micrometeoroids in the lunar environment. Nor was the analysis of the irregular gravity field published. In addition to scientific instruments, the spacecraft carried technology tests of lubricants for operating gears and bearings in vacuum to qualify them for use on lunar rovers.
Ye-6LS Luna 14:
1. Lunar communications system test
2. Cosmic ray detector
3. Solar wind plasma sensors
4. Radiation dosimeter
5. Gravitational field mapping experiment (using spacecraft tracking)
6. Lunar rover wheel drive technology experiment
The main goal of Luna 14 was to test the spaceborne and ground segments of the new communication system for the manned lunar program. Other objectives were to continue to investigate the lunar radiation and plasma environment and to use a new: navigation system to more precisely map the lunar gravity field and librations. The spacecraft also carried more engineering tests of lunar rover motors including gears, ball bearings and lubricants Гог vacuum operation.
Mission description:
On March L 1966, the first spacecraft, Yc-6S No.204. was stranded in parking orbit when the fourth stage lost roll control during the unpowered coast and was unable to lire its engine for the escape burn. It was designated Cosmos 111 and re-entered the atmosphere 2 days later.
Four weeks later on March 31,1966. Ye-6S No.206 was successfully dispatched as Luna 10. It made a mideourse correction the following day and then at 18:44 IJT on April 3 it became the first spacecraft to enter orbit around another celestial body, achieving a 350 x 1,017 km orbit inclined at 72 degrees to the lunar equator with a period of 2 hours 58 minutes. The cruise stage then released the or biter module. On April 4 the ‘Internationale was played to the 23rd Congress of the Communist Party of the Soviet Union. In Tact w hat the Congress heard was a rehearsal playback from the previous evening, because at a second rehearsal on the morning of the meeting it w as observed that one of the notes had gone missing. Luna 10 operated for 56 days. 460 lunar orbits and 219 radio transmissions before the battery drained and contact was lost on May 30, 1966.
Spacecraft Ye-6LF No. 101 was launched on August 24, 1966, as Luna 11, and at 21:49 UT on August 28 it entered a 160 x 1,193 km lunar orbit that was inclined at 27 degrees with a period of 3 hours. Coming 2 weeks after the first US or biter, there was every expectation in the West that the Soviet mission would send back images. The transmissions had been recoded to prevent interception by the likes of Jodrell Bank, but no pictures w’ere forthcoming. An attitude control thruster had failed and prevented aiming either the camera or the ultraviolet instrument at the lunar surface. It was suspected that something had become lodged in the nozzle of the thruster. By sheer bad luck, the x-ray and gamma-ray spectrometers also failed. The spacecraft w as placed into a spin stabilized mode and the other experiments apparently worked satisfactorily. After 38 days, 277 orbits and 137 radio transmissions the batteries ran out on October 1, 1966, and the Soviets, without mentioning imaging, reported that the mission was complete.
Ye-6LF No. 102. Luna 12. entered a 3 hour 25 minute 103 x 1,742 km lunar orbit inclined by 36.6 degrees on October 25, 1966. It did not suffer the problems of its predecessor except for the ultraviolet spectrometer, which again failed. Tile primary objectives were the lunar photography that Luna 11 had been unable to provide and to continue to chart the gravitational field. On October 29 the spacecraft transmitted its first photographs. In this regard the Soviets were 2 months behind the Amerieans. A total of 40 images were returned by each of the two cameras. Once the spacecraft had finished imaging, it was placed into a spin stabilized mode and the other tasks were sueeessfully performed, ineluding testing electric motors for a rover, hven with the new gravity maps developed by tracking Luna 1L the orbit of Luna 12 deviated surprisingly far from that predicted. Its perilune dropped by 3 to 4 km, day relative to the prediction, and the failure of one of the attitude control thrusters made it difficult to raise the perilune to compensate. Finally, on January 19, 1967. after 85 days. 602 orbits and 302 communication sessions, transmissions ceased.
The next mission to be launched was a test flight of the second modification to the orbiter. The Ye-6LS No. lll spacecraft was to be launched into a highly elliptical Earth orbit with an apogee near 250.000 km in order to perfect a means of accurately measuring and adjusting an orbital trajectory to compensate for gravity anomalies, but the fourth stage shut down prematurely leaving the spacecraft in a lower 260 x 60,710 km orbit. Despite the low apogee, it was probably put through its intended operations. Designated Cosmos 159, it re-entered on November 11, 1967. The Ye – 6LS No. l 12 spacecraft failed to achieve parking orbit when the third stage ran out of propellant early at the 524 second mark as a result of excessive fuel consumption through the turbine gas generator. The last spacecraft of this type. Yc-6LS No. l 13. was successfully dispatched as Luna 14 and at 19:25 UT on April 10. 1968. it entered a 160 x 870 km lunar orbit at 42 degrees. It marked the end of the second generation of Luna missions.
Results:
Luna 10 conducted an extensive study of the Moon from lunar orbit. Its path varied much more than the Soviets expected. This was due to a very uneven gravity field that featured localized ‘mass concentrations’ (mascons) below the surface. Luna 10 established the importance of charting the lunar gravity field, and also of providing spacecraft with robust propulsion for precise control of their orbital trajectories. The Soviets discovered this well before the Americans, who were generally given credit because their space program results were more widely published. Luna 10 found the Moon to have no detectable atmosphere, the lunar surface to have large expanses of basalt but few, if any. granitic provinces, and measured the amount of potassium, uranium, and thorium in the crust. It mapped a magnetic field whose strength was 0.001 % of Earth, and found it not likely to be intrinsic. It showed that the Moon had no trapped radiation belts like those of Earth. The micrometeoroid flux and cosmic radiation in lunar orbit was also measured.
The x-ray and gamma-ray spectrometers on Luna 11 failed and imaging was not possible, but it was able to make observations of solar radiation and also conducted a successful test of lunar rover reduction gear operation in vacuum. The first images
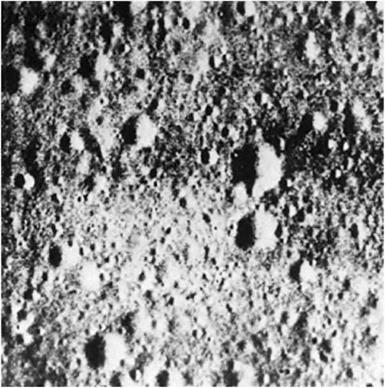
Figure 10.12 Luna 12 image taken from 250 km of an area of 25 square kilometers south of the crater Aristarchus.
|
were obtained by Luna 12. On October 29, 1966, it transmitted images of the Sea of Rains and the crater Aristarchus with resolution of 15 to 20 meters. Owing to their low quality, only the first few images were released. Ironically, the Soviets used the more extensive and far better imagery from the Lunar Orbitcr scries to select landing sites. Furthermore, like the Americans they ultimately chose three sites, one each in the Sea of Tranquility, the Central Bay and the Ocean of Storms. The radio tracking of Luna 11 and 12 revealed the need for even better orbital tracking experiments to provide a more precise lunar gravity map. Luna 12 also detected x-ray fluorescence of the surface induced by the solar wind, and this provided a means of measuring ihe composition of the surface. Radiation fields and micrometeoroid flux were measured in lunar space and the engineering test of reduction gears was successful.
The mission of Luna 14 passed almost without comment, perhaps because to have described what it was doing would have revealed too much about the manned lunar program. It was assumed in the West at the time to be a failed photographic mission, but it is now known to have mapped the figure of the Moon and its gravity field to a high degree of precision, to have provided data on the propagation and stability of radio communications to the spacecraft at different orbital positions, measured solar wind plasma and cosmic rays in lunar orbit, measured the librational motion of the Moon, and determined the Harih-Moon mass ratio. The eommunicalions system for the manned lunar program was also successfully tested. Further engineering tests of rover motors were conducted to select the materials and lubricants that operated best in vacuum for the systems intended for roving vehicles.