Campaign objectives:
The Ye-8 series was developed to support the Soviet lunar cosmonaut program. By the time that the Soviets entered the Moon race in mid-1964 Russian engineers at OKB-1 had already developed plans for a lunar rover. This, along with all the other lunar robotic programs, was transferred to NPO-Lavoehkin in 1965. In early 1966 an automated lunar surface rover entered the mission plan for supporting a cosmonaut on the lunar surface. The function of the rover was to precede the cosmonaut to the landing site, to survey and certify the site as safe for landing, to act as a radio beacon to guide the manned lander in, to inspect this lander after touchdown and certify it as safe for ascent, and, if it were not so, to transport the cosmonaut to a backup ascent vehicle that was already in place.
When the robotic lunar exploration program was transferred to NPO-Lavoehkin. Georgi Babakin set to work on a design for a spacecraft to meet these requirements. The availability of the powerful four-stage Proton launch vehicle using the Block D translunar injection stage enabled the resulting Ye-8 to be much heavier and more complex than its Ye-6 predecessor. The multi-purpose, in-line module design of the Ye-6 series was abandoned for a spacecraft design suited principally for soft landing a rover, and eventually other types of payload.
Spacecraft:
The spacecraft comprised three main components; a lander stage on the bottom, the rover that was carried on top, and a pair of side-mounted backpacks’, each of which had avionics and two cylindrical propellant tanks.
Spacecraft launched
|
|
First spacecraft:
|
Ye-8 Ко.201
|
Mission Type:
|
Lunar Lander and Rover
|
Country і Builder:
|
USSR NPO-Lavochkin
|
Launch Vehicle:
|
Proton-K
|
Launch Date ‘: 7 ime:
|
February 19, 1969 at 06:48:15 UT (Baikonur)
|
Outcome:
|
Shroud failure, vehicle disintegrated.
|
Second spacecraft:
|
Luna 17 (Yc-8 No.203)
|
Mission Type:
|
Lunar Lander and Rover
|
Country і Builder:
|
USSR NPO-Lavochkin
|
Launch Vehicle:
|
Proton-K
|
Launch Date; Time:
|
November 10, 1970 at 14:44:01 UT (Baikonur)
|
Lunar Orbit Insertion:
|
November 15, 1970
|
Lunar Landing:
|
November 17. 1970 at 03:46:50 UT
|
Mission End:
|
September 14. 1971 at 13:05 UT
|
Outcome:
|
Success.
|
Third spacecraft:
|
Luna 21 (Ye-8 No.204)
|
Mission Type:
|
Lunar Lander and Rover
|
Country і Builder:
|
USSR NPO-Lavochkin
|
Launch Vehicle:
|
Proton-K
|
Launch Date ‘: I ime:
|
January 8, 1973 at 06:55:38 UT (Baikonur)
|
Lunar Orbit Insertion:
|
January 12, 1973
|
Lunar Landing:
|
January 15, 1973 at 22:35 UT
|
Mission End:
|
June 3, 1973
|
Outcome:
|
Success.
|
Cruise and lander stages:
The lander stage was based on a quartet of 88 an diameter spherical propellant tanks arranged in a square 4 meters on a side and connected using cylindrical inter-tank sections. These tanks fed a single engine whose thrust could be varied over the range 7.4 to 18.8 kN and a set of six vernier engines, two of which were mounted next to the main engine and were for use during the final descent to the surface. The other verniers were positioned around the periphery to provide stabilization. The landing system, engine, and radar altimeter were located between the tanks on the underside of the square tank assembly. Hach of the tanks supported a shock absorbing landing leg. Attitude control thrusters were located at various places around the lander. The avionics and attitude control sensors to control the translunar trajectory, lunar orbit insertion, orbital maneuvers, and landing, were housed in the inter-tank cylindrical sections. Water cooling was used for thermal control. Communications at 922 MHz and 768 MHz were by way of a cone-shaped antenna mounted on a boom. Uplink was at 115 MHz.
-Figure 11,3 Luna 17 spacecraft diagram (from Ball et al.) and during test at Lavochkin.
|
|
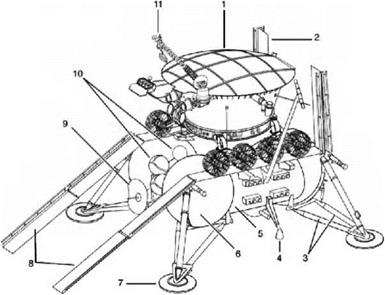
Figure 11.4 Luna 17 lander (by James Garry): 1. Lunokhod rover; 2. Folded exit ramp; 3. Shock absorbers; 4. Steering rockets; 5. Service module and avionics; 6. Propulsion tank; 7. Landing foot; 8. Extended exit ramps; 9. Radio altimeter; 10. Attitude control gas tanks; 11. Conical low-gain antenna and steerable directional helical antenna.
|
|
The two detachable backpacks’ were mounted vertically on opposite sides of the square tank assembly and were for cruise and orbital operations, bach consisted of a pair of 88 cm diameter cylindrical tanks, between which were avionics and battery modules. The tanks contained propellants to feed the main engine. On top of each of these tanks was a smaller spherical tank of nitrogen for the cold gas attitude control system.
The Isayev design bureau built the new throttleable KTDIJ-417 main engine. Its purpose was to conduct midcourse maneuvers during the translunar coast, lunar orbit insertion, orbital maneuvers, and key portions of the descent. Once the operational orbit at about 100 km altitude had been achieved, descent to the surface began with a burn of about 20 m/s to lower the perilune to about 15 km directly over the landing site. The backpacks were jettisoned and. with perilune looming, the main engine was ignited for a 1.700 m s ‘dead stop’ burn lasting 270 seconds designed to completely eliminate its horizontal velocity. After the spacecraft had free fallen to an altitude of about 600 meters and accelerated in the weak lunar gravity to a descent rate of about 250 m/s the main engine was reignited. This was shut down at 20 meters and the landing verniers ignited until a contact switch cut them off at a height of 2 meters. If all had gone to plan, the vehicle would then touch down at a velocity not exceeding 2.5 m/s. Unlike the Ye-6 soft landers, whose targets were constrained by the need to make a vertical descent from the translunar coast, the new spacecraft, by first going into orbit, could land anywhere.
f or a rover mission, two sets of folding ramps were mounted on top of the upper side of the lander fore and aft of the rover, whose wheels were on the middle of the ramps. The ends of the ramps were carried folded up against the rover, and once on the Moon they were unfolded and lowered to provide the rover with two options for driving off the lander down onto the surface.
Lunokhod rover:
The body of the Lunokhod rover was a tub-like pressurized magnesium alloy shell for avionics, instruments and environmental controls covered by a large hinged lid. In daylight on the lunar surface the convex lid would be opened over the rear of the rover to expose solar cells on the inside surface of the lid to generate pow er and also to expose radiators in the top of the ‘tub’ for thermal control. In darkness the lid was closed. It was a very simple and effective design. The solar cells (Si on Lunokhod 1 and GaAs on Lunokhod 2) gave 1 kW of power to recharge the internal batteries. The body was mounted on a carriage of eight wheels, 51 cm in diameter and made of ware mesh with titanium blade treads. This design was in response to the data on lunar soil provided by Luna 9; the thin dust layer and firm soil that this found led to the abandonment of a caterpillar track design. Each wheel had its own suspension system using a special fluoride based lubricant to operate in vacuum, a pressurized independent DC motor and an independent brake.
The rover was controlled entirely from Earth by a five-person team, there was no automated mode, and steering required independently changing the speed settings on the wheels. It could move with only two operational wheels on each side, and any of
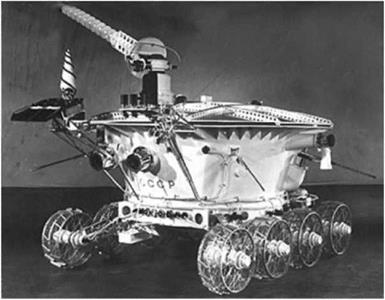
Figure 11.5 Lunokhod 1.
|
the axles could be severed to shed a wheel if it became locked. The smallest turning radius was 80 cm. Internal gyroscopes indicated its orientation. It was designed to drive over obstacles 40 cm high or 60 cm wide, to climb slopes of 20 degrees, and to maneuver on slopes as steep as 45 degrees. There were fail-safe devices to prevent movement over excessive slopes. Lunokhod 1 had only one driving speed, 800 m/hr, traveling either forward or in reverse, but Lunokhod 2 was capable of 800 and 2,000 m/hr in either direction.
The control team operated and navigated the vehicle by viewing through a pair of television cameras mounted on the front of the rover. These returned low resolution images at a rate of 20 seconds/frame for Lunokhod 1 and at the much improved rate of up to 3.2 seconds/frame for Lunokhod 2. The signal time delay to the Moon and back to Earth was 5 seconds, which had an effect on operations. Pour other scanning photometer imagers of the type used on Luna 9 were mounted on the chassis. A pod on each side held a vertically mounted imager to give a 180 degree view at a 15 degree down angle, jointly providing a full panoramic view around the rover. A second imager was set above the first, nearer the top of the ‘tub’, and was mounted horizontally. These would jointly provide a full vertical panorama that included the sky and stars for navigation at the zenith and a vehicle level indicator at the nadir.
The rover was designed to survive three lunar nights, each lasting a fortnight over a period of 3 months. In darkness it was the kept alive by a small radioisotope heater with 11 kg of polonium-210 and by a radiator on top of the closed lid. Thermal control was by circulating internal air and by open-cycle water cooling. The rover was equipped with a conical low gain antenna, a steerable directional helical high gain antenna, television cameras, and extendable devices to impact the surface for soil density and mechanical property tests. Lunokhod 1 was 135 cm high, 170 cm long, 215 cm wide at the top, 160 cm wide at the wheels, had a wheelbase of 2.22 x
1.6 meters, and a mass of 756 kg.
Lunokhod 2 was an improvement based on experience with Lunokhod L It had an additional camera on the front at adult height for easier navigation. Its images could be transmitted at rates of 3.2, 5.7, 10.9 or 21.1 seconds‘frame, with the fastest rate being instrumental in improving driving operations. The 8-wiiecl drive system was improved, and Lunokhod 2 was twice as fast for twice the range. Additional science instruments were carried.
Luna 17 launch mass: 5,660 kg (landed mass 1,900 kg; rover 756 kg)
Luna 21 launch mass: 5,700 kg (landed mass 1,836 kg; rover 836 kg)
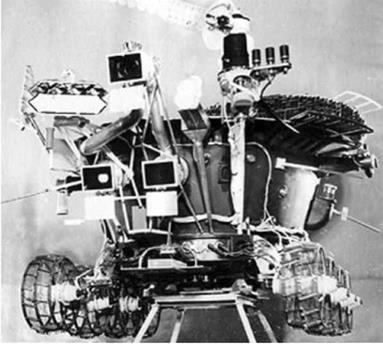
Figure 11.6 Lunokhod 2.
|
Payload:
Lunokhod 1:
1. Two television cameras for stereo images in the direction of travel
2. Four panoramic imagers
3. PrOP odometer/speedometer and soil mechanics penetrometer
4. Soil x-ray fluorescence spectrometer
5. Cosmic ray detectors
6. X-ray telescope for solar and extragalactic observations
7. Laser retro-reflector (France)
8.
Radiometer
four imagers were facsimile cameras of the type flown on Luna 9, with improved sensitivity and gain control and mounted two to a side. One camera in each pair was mounted for 180 degree horizontal scanning and the other for vertical scanning from surface to sky. bach 180 degree panorama consisted of 500 x 3,000 pixels. Between them, each pair of cameras provided a 360 degree panorama. The horizontal ones provided context for the forward cameras and the vertical ones assisted navigation in the driving process.
A ninth spiked wheel trailed behind the rover with an odometer to measure distance and speed. The surface penetrometer was mounted on a pantograph. The French laser retro-reflector weighed 3.5 kg and consisted of fourteen 10 cm silica glass prisms. It was designed for 25 cm accuracy. Due to Soviet secrecy, the French were given only a drawing for how the device would be mounted and were not told in advance what kind of lunar vehicle would carry it.
Lunokhod 2:
1. Three front television cameras for stereo images in the direction of travel
2. Four panoramic imagers
3. PrOF odometer/speedometer and soil mechanics penetrometer
4. Soil x-ray fluorescence spectrometer (Rifma-M)
5. Cosmic ray detectors
6. X-ray telescope for solar and extragalactic observations
7. Laser retro-reflector (France)
8. Radiometer
9. Visible-ultraviolet photometer
10. Boom magnetometer
Based on experience with Lunokhod 1, a third forward viewing television camera was mounted higher on Lunokhod 2 to provide a better driving perspective while the lower pair of television cameras provided stereo images of potential obstacles. The visible-ultraviolet photometer was to detect Larth airglow and galactic ultraviolet sources. The magnetometer was mounted on a 1.5 meter long boom in front of the rover. A Soviet-made photocell was added to the French retro-reflector to register laser strikes and the x-ray fluorescence spectrometer was improved.
Mission description:
First attempt falls do war aitge
The first attempt to launch a Ye-8 with a rover failed spectacularly on February 19. 1969, when the payload stack on top of the vehicle disintegrated 51 seconds into the flight. The launcher then exploded and scattered wreckage 15 miles downrange. The investigation discovered that at the point of maximum dynamic pressure, when the loads on the vehicle were greatest, the newly designed payload shroud for the Proton failed. The radioisotope heater that was to have kept the rover warm during the lunar night was never recovered from the debris, and rumors persist that the soldiers who actually found it decided to use it to heat their barracks during that year’s very cold winter.
Luna 17
A second attempt to launch a lunar rover was not made until 20 months later. After the loss of the first mission, the Ye-8 program had focused on attempts at automated lunar sample return in an effort to upstage Apollo. After Luna 16 succeeded with a returned sample in October 1970 it was decided, to launch a rover next. The back to back successes of an automated sample return mission and. a rover one month apart were impressive milestone achievements for the Soviet robotic lunar program.
Luna 17 was launched on November 10, 1970. After midcourse corrections on the 12th and 14th, it entered an 85 x 141 km lunar orbit on the 15th inclined at 141 degrees with a period of 115 minutes. It lowered its perilune to 19 km on the 16th and then at 03:46:50 UT on the 17th successfully touched down at a speed of about 2 m/s in the Sea of Rains at 38.25°N 325.00°E.
The Soviets announced their fourth lunar soft landing, and Westerners expected another sample return like Luna 16. However, about 3 hours after landing, at 06:28 UT, the ramps were lowered, the camera covers released, pictures of the ends of the
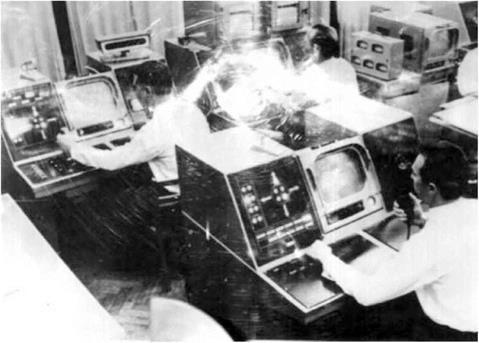
Figure 11.8 Lunokhod 1 operations crew.
|
ramps were taken to ensure that there were no obstructions, and Lunokhod 1 rolled down the front ramp and 20 meters across the surface. The next day it remained in place and recharged its batteries, then it traveled 90 and 100 meters on the next two days. On the fifth day, 197 meters from its lander, it closed its lid and shut down for the forthcoming lunar night.
The public reaction across the world was astonishing. Somehow, people resonated strongly with the idea of a robotic rover driving around on another world, even if the experience was only a virtual one. The Luna 17 rover was a triumph heralded by the Soviet and Western press alike, whereas the Luna 16 sample return had only gained fleeting admiration. The appeal of the Lunokhod may have been partly derived from its physical form. Its antics were followed ardently in the press for the first few’ days, and the coverage would probably have continued were it not for the requirement to
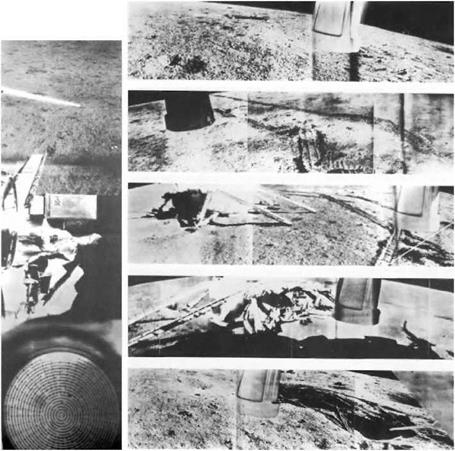
Figure 11.9 A set of Lunokhod 1 horizontal panoramas taken during its return to the landing site and vertical panorama from nadir to horizon while still mounted on the lander before deployment.
|
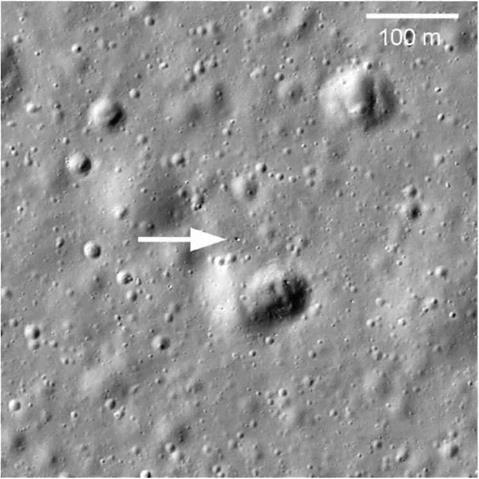
Figure 11.10 Lunokhod 1 on the surface from NASA’s Lunar Reconnaissance Orbiter.
|
shut it down for the long lunar night. It would be more than a quarter of a century before the US would recreate the excitement of a robotic rover on another world.
Lunokhod 1 survived its first lunar night and continued its activities. The drivers had some difficulty coming to terms with the frame rate of 20 seconds, and it was realized that the driving cameras had been set too low on the vehicle because their perspective was more like sitting on a chair than standing upright. And their images were so overexposed that the contrast in the scene was poor, especially near lunar noon. Initially excluded from the control room, the scientists had difficulty m having the rover pause at interesting rocks. This was because the engineers’ measure of success was distance covered. However, as the mission wore on it became easier for the scientists to achieve their objectives.
The operators drove the rover over 197 meters on the first lunar day, and as far as 2 km on the fifth lunar day. To test its navigational system, on one early excursion it returned to the lander stage. Over a period of 10 months it traversed rough hills and valleys and crossed many craters. It survived the -150°C cold of the lunar night and 100 C heat of lunar noon. It twice became stuck in craters, but after some effort was able to extract itself. The drivers had difficulty navigating because of the low mount of the cameras which meant they often did not spot a crater until the last moment. At noon the lack of shadows reduced the contrast to /его, making steering impossible. The rover survived a solar flare that might have been fatal for cosmonauts and an eclipse during which it was temporarily plunged into darkness. On the tenth lunar day it was spotted from orbit by the Apollo 15 astronauts.
The last successful communications session with Lunokhod 1 ended at 13:05 UT on September 14. 1971, after the internal pressure suddenly dropped. Officially, the mission concluded on October 4, 1971, the 14th anniversary of Sputnik. Fortunately, during its last communication cycle it had been parked with the laser retro-reflector in a position where it could continue to be used. Lunokhod 1 exceeded its expected lifetime of three lunar days by functioning for eleven lunar days. It traveled a total of 10,540 meters and transmitted more than 20.000 individual pietures, 206 panoramas. 25 x-ray elemental soil analyses, and more than 500 soil penetrometer tests. It was a spectacular success.
Luna 21
The next rover was modified to take account of the lessons from Lunokhod 1. and on January 8, 1973. Luna 21 was launched carrying Lunokhod 2. It performed a midcourse maneuver the next day, and on January 12 entered a 90 x 110 km lunar orbit inclined at 62 degrees with a period of 118 minutes. It lowered its perilune to 16 km the following day and then on January 15 fired its main engine at perilune to deorbit itself. At an altitude of 750 meters the main engine ignited again to slow7 the rate of descent. At 22 meters this engine was shut down. The verniers took over to a height of 1.5 meters and were cut off. After falling the remaining distance the 7 m/s shock was absorbed by the legs. Luna 21 landed at 23:35 IJT at 26.92 N 30.45 E in Le Monnier bay. an eroded and lava flooded crater cut into the Taurus Mountains on the eastern shore of the Sea of Serenity.
The Lunokhod 2 rover immediately took TV images of the surrounding area from its perch atop the lander. After rolling down onto the surface at 01:14 UT on January 16 it took pictures of the lander and the landing site. It remained in place for 2 days until its batteries were charged, then took some more pictures and began its traverse. During its first full lunar day it covered a greater distance than its predecessor had in eleven lunar days. In one day. it traveled as much as 1.148 meters. It climbed a hill 400 meters high and photographed the peaks of the Taurus mountains poking over the horizon with Earth in the sky above. In late January 1973 an American scientist attending an international conference on planetary exploration in Moscow^ gave a set of Apollo 17 photos of the area w’here Luna 21 landed to Russian scientists at the meeting. These highly detailed photographs were used to navigate Lunokhod 2 to a rille some distance east of its landing site.
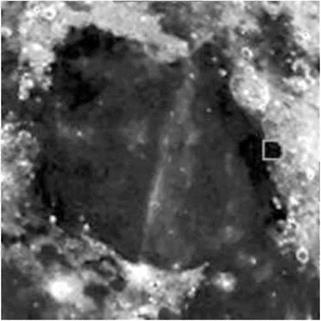
Figure 11.11 Lunokhod 2 site in the Sea of Serenity.
|
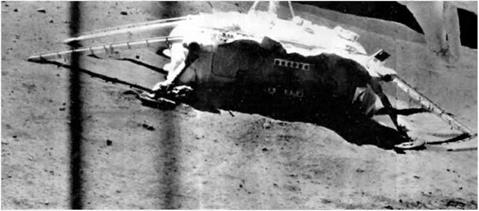
Figure 11.12 Picture of lander from Lunokhod 2.
|
Rover operations were conducted during the lunar day, stopping occasionally to recharge the battery using its solar panels. It would hibernate during the lunar night, using the radioisotope heater to maintain thermal control.
Lunokhod 2 operated lor about 4 months, drove 37 km over terrain including hilly upland areas and rilles, more than four times the area of its predecessor, and returned over 80,000 individual images and 86 panoramas. It made hundreds of elemental analyses and mechanical tests of the soil, as well as being used for laser
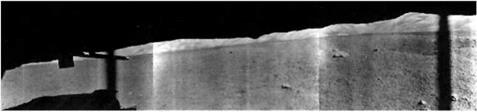
Figure 11.13 Lunokhod 2 panorama around the landing area with Taurus Mountains in the distance.
|
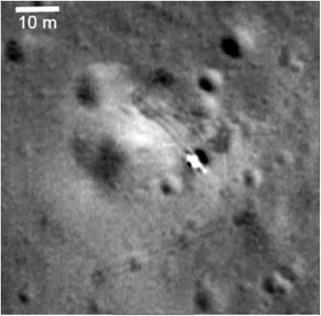
Figure 11.14 Luna 21 lander on the surface from NASA’s Lunar Reconnaissance Orbitcr showing rover tracks.
|
ranging and other experiments. On May 9, 1973, it accidentally rolled into a small 5 meter crater whose depth had been concealed by a shadow. As the rover was backing itself out, it scraped its lid on the crater wall, causing a spray of dust to cover the solar panel. When the lid was closed for the lunar night, this soil was dumped onto the radiators. On opening the lid for the next lunar day, the resulting thermal and power problems led to the vehicle’s demise, which was announced on June 3.
Results:
Significant scientific results derived from analyzing the pictures of rocks and soil, wheel tracks, craters and other geological features observed by the twjo Lunokhods in more than 20,000 single frame images and 200 panoramas. There were many soil mechanics measurements by the penetrometer and chemical analysis results from the x-ray fluorescence spectrometer. The Sea of Rains and floor of Le Monnier proved to be a typical mare basalt, but the uplands around Le Monnier (the surviving part of the rim of the eroded crater) turned out to have higher concentrations of iron, silicon, aluminum and potassium.
Lasers fired by the French from the Pic du Midi observatory and by the Russians from the Semeis observatory in the Crimea used the retro-reflectors to determine the distance to the Moon to within 3 meters for Lunokhod 1, and 40 cm for Lunokhod 2. In the long term, such observations established the periodic and secular dynamics of the Moon. The cosmic ray instruments recorded the radiation on the Moon, and the x – ray telescope observed the Sun and the galaxy. The magnetometer on Lunokhod 2 measured a very weak magnetic field with variations due to currents induced by the interplanetary magnetic field. The photometer made some surprising observations of the brightness of the lunar sky. In particular, it determined that the day-time lunar sky was contaminated with some dust, and in Earthlight the night-time lunar sky was 15 times brighter than the sky on Earth at full Moon; findings which did not bode well for one day establishing astronomical observatories on the lunar surface.