In contrast to STOL aircraft systems, which used wing lift generated by forward movement to take off, VTOL aircraft would necessarily have to have some provision for direct vertical propulsive thrust, with the thrust level well in excess of the airplane’s operating weight, to lift off the ground. This drove deflected propeller thrust, tilt wing, tilt rotor, and vectored jet thrust technical approaches, all of which NASA researchers intensively studied. In all of this, the researchers’ assessment of the system’s VTOL control capability was of special interest—for they had to be able to be controlled in pitch, roll, and yaw without any reliance upon the traditional forces imposed upon an airplane by its movement through the air. The first two approaches that NACA-NASA researchers explored were those of deflected propeller flow and pivoted tilt wings.
At the beginning of 1958, the Ryan Company of San Diego unveiled its Model 92, the VZ-3RY Vertiplane. The Vertiplane, a single-seat twin – propeller high wing design with a T-tail, used propeller thrust to attain vertical flight and maintain hover, deflecting the propeller slipstream via a variable-area and variable-camber wing. The wing’s trailing edge consisted of large, 40-percent-chord, double-slotted flaps that transformed into a gigantic curved flow channel, with wingtip ventral fins serving to further entrap the air and concentrate its flow vertically below the craft. Roll control in hover came via varying the propeller pitch to achieve changes in slipstream flow. Power to its twin three-bladed propellers was furnished by a single Lycoming T53 turboshaft engine, which also had its exhaust channeled through a tailpipe to a universal-joint nozzle that furnished pitch and yaw control for the airplane when it was in hover mode via deflected jet thrust.[1382]
Before the aircraft flew, Ames researchers undertook a series of wind tunnel tests in the 40-foot by 80-foot full-scale wind tunnel to define performance, stability and control, and handling and control characteristics.[1383] As a result of these tests, the aircraft’s landing gear was changed from a "tail-dragger” to tricycle arrangement, and engineers added a ventral fin to enhance directional stability in conventional flight. Thus modified, the VZ-3RY completed its first flight January 21, 1959, piloted by Ryan test pilot Pete Girard. Less than a month later, it
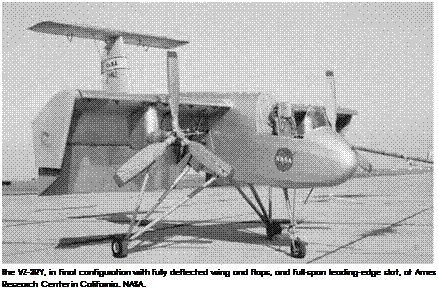 |
 |
was damaged in a landing accident at the conclusion of its 13th flight, when a propeller pitch control mechanism malfunctioned, leaving the VZ-3RY with insufficient lift to drag (L/D) available to flare for landing. It was late summer before it returned to the air, being delivered to Ames in 1960 for NASA testing. Howard L. Turner oversaw the project, and Glen Stinnett and Fred Drinkwater undertook most of the flying. The aircraft was severely damaged when Stinnett ran out of nose-down control at a low-power setting and the aircraft pitched inverted. Fortunately, Stinnett ejected before it nosed into the salt ponds north of the Moffett Field runway. Despite this seemingly disastrous accident, the aircraft was rebuilt yet again and completed the test program. The addition of full-span wing leading-edge slats to enhance lift production permitted hover out of ground effect (OGE). However, air recirculation effects limited in ground effect (IGE) operation to speeds greater than 10 knots, as marginal turning of the slipstream and random upset disturbances caused by slipstream recirculation prevented true VTOL performance. A static pitch instability was often encountered at high lift coefficients, and large pitch trim changes occurred with flap deflection and power changes. The transition required careful piloting technique to avoid pitch – up. Although adequate, descent performance was limited in the extreme by low roll control power and airflow separation on the wing when power was reduced to descend. Despite these quirks and two accidents,
the VZ-3RY demonstrated excellent STOL performance, achieving a maximum lift coefficient of 10, with a moderate to good cruise speed range. Thus, it must be considered a successful research program. Transitions were completed from maximum speed down to 20 knots with "negligible change in longitudinal trim and at rates comparable to those done with a helicopter.”[1384] Indeed, as Turner and Drinkwater concluded in 1963, "Flight tests with the Ryan VZ-3RY V/STOL deflected-slipstream test vehicle have indicated that the concept has some outstanding advantages as a STOL aircraft where very short take-off and landing characteristics are desired.”[1385] As well as pursuing the BLC and deflected slipstream projects, NASA researchers examined tilt wing concepts then being pursued in America and abroad. The tilt wing promised a good blend of moderate low – and high-speed compatibility, with good STOL performance provided by slipstream-induced lift. For takeoff and landing, the wing would pivot so that the engine nacelles and propellers pointed vertically. After takeoff, the wing would be gradually rotated back to the horizontal, enabling conventional flight. Various research aircraft were built to investigate the tilt wing approach to V/STOL flight, notably including the Canadair CL-84, Hiller X-18, the Kaman K-16B, and the joint-service Ling-Temco – Vought XC-142. The first such American aircraft was the Boeing-Vertol VZ-2 (the Vertol Model 76). It was powered by a single Lycoming YT53L1 gas turbine, driving two propellers via extension shafts and small tail fans for low-speed pitch and yaw control. Conceived from a jointly funded U. S. Army-Office of Naval Research study, the VZ-2 first flew in August 1957 and was an important early step in demonstrating the potential of tilt wing V/STOL technology. On July 16, 1958, piloted by Leonard La Vassar, it made the world’s first full-conversion of a tilt wing aircraft from vertical to horizontal flight, an important milestone in the history of V/STOL. Vertol completed its testing in September 1959 and then shipped the VZ-2 to Langley Research Center for evaluation by NASA.[1386]
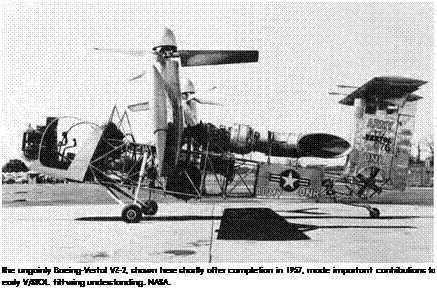 |
 |
Subsequent Langley tests confirmed that the tilt wing was undoubtedly promising. However, like many first-generation technological systems, the VZ-2 had a number of limitations. NASA test pilot Don Mallick recalled, "it was extremely difficult to fly,” with "lots of cross-coupling between the roll and yaw controls,” and that "It took everything I had to keep from ‘dinging’ or crashing the aircraft.”[1387] Langley research pilot Jack Reeder found that its VTOL roll control—which, as in a helicopter, was provided by varying the propeller pitch and hence its thrust— was too sensitive. Further, the two ducted fans at the tail responsible for pitch and yaw control furnished only marginal control power. In particular, weak yaw control generated random heading deviations. When slowing into ground effect at a wing tilt angle of 70 degrees, directional instabilities were encountered, though there was no appreciable aerodynamic lift change.[1388]
Reflected flows from the ground caused buffeting and unsteady aircraft behavior, resulting in poor hover precision. Because of low
pitch control power, lack of a Stability Augmentation System (SAS), and low inherent damping of any pitch oscillations, researchers prudently undertook hover trials only in calm air. Among its positive qualities, good STOL performance was provided by slipstream-induced lift. Transition to wing-supported flight was satisfactory, with little pitch – trim change required. In transitions, as the wing pivoted down to normal flight position, hover controls were phased out. The normal aerodynamic controls were phased in, with the change from propeller to wing – supported flight being judged satisfactory. However, deceleration on descent was severely restricted by wing stall. When power was reduced, lateral – directional damping decreased to unsatisfactory levels. Changes were made to "droop” the leading edge 6 degrees to improve descent performance, and the modification improved behavior and controllability so greatly that Langley test pilot Jack Reeder concluded the "serious stall limitations in descent and level-flight deceleration were essentially eliminated from the range of practical flight operation, at least at incidence angles up to 50°.”[1389] In spite of this seemingly poor "report card,” the awkward – looking VZ-2 contributed greatly to early understanding of the behavior and foibles of V/STOL tilt wing designs. All together, it completed 450 research sorties, including 34 full transitions from vertical to horizontal flight. The VZ-2 flight program proved to be one of the more productive American V/STOL programs, furnishing much information on wing- propeller aerodynamic interactions and basic V/STOL handling qualities.[1390]
In addition to the pioneering VZ-2, the Hiller and Kaman companies also pursued the concept, the former for the Air Force and the latter for the Navy, though with significantly less success. Using an off – the-shelf development approach followed by many V/STOL programs, Hiller joined the fuselage and tail section of a Chase YC-122 assault transport to a tilt wing, creating the X-18, the first transport-sized tilt wing testbed. It used two Allison T40 turboprop engines driving three-bladed contra-rotating propellers, plus a Westinghouse J34 to furnish pitch control via a lengthy tailpipe. The sole X-18 made a conventional flight in November 1959 and completed a further 19 test sorties before being
grounded. Though it demonstrated wing tilt in flight to an angle of 33 degrees, it never completed a VTOL takeoff and transition. On November 4, 1960, a propeller malfunction led to it entering an inverted spin. Through superb airmanship, test pilots George Bright and Bruce Jones recovered the aircraft and landed safely, but it never flew again.[1391] Kaman undertook a similar development program for the Navy, joining a tilt wing with two General Electric T58-GE-2A turboshaft engines to the fuselage and tail section of a Grumman JRF Goose amphibian, creating the K-16B. Tested in Ames’s 40-foot by 80-foot tunnel, the K-16B never took to the air.[1392]
Despite these failures, confidence in the tilt wing concept had advanced so rapidly that in February 1961, after 2 years of feasibility studies, the Department of Defense issued a joint-service development specification for an experimental VTOL transport that could possibly be developed into an operational military system. After evaluating proposals, the department selected the Vought-Hiller-Ryan Model VHR 447, ordering it into development under the Tri-Service Assault Transport Program as the XC-142A.[1393] All three of these companies had previously employed variable position wings, with the F-8 Crusader fighter, the X-18, and the VZ-3RY, though only the last two were V/STOL designs. The XC-142A was powered by four General Electric T64 turboshaft engines, each rated at 3,080 horsepower, driving four-bladed Hamilton Standard propellers, with the propellers cross-linked by drive shafts to prevent a possibly disastrous loss of control during VTOL transitions. The combination of great power and light weight ensured not only that it could take off and land vertically, but also that it would have a high top-end speed of over 400 mph. Piloted by Stuart Madison, the first of five XC-142As completed a conventional takeoff in late September 1964, made its first hover at the end of December 1964, and accomplished its first transition from vertical to horizontal flight January 11, l965, "with no surprises.”[1394]
The five XC-142A test aircraft underwent extensive joint – service evaluation, moving a variety of vehicles and troops, undertaking
simulated recovery of downed aircrew via a recovery sling, landing aboard an aircraft carrier, and even flying a demonstration at the 1967 Paris Air Show. With a payload of 8,000 pounds and a gross weight of 37,500 pounds, the XC-142A had a thrust-to-weight ratio of 1.05 to 1. In STOL mode, with the wing set at 35 degrees and with flaps set at 30 degrees, the XC-142A could almost double this payload yet still clear a 50-foot obstacle after a 200-foot takeoff run.[1395] Unfortunately, program costs rose from an estimated $66 million at inception to $115 million (in FY 1963 dollars), resulting in overruns that eventually truncated the aircraft’s development.[1396] The five aircraft experienced a number of mishaps, most related to shafting and propulsion problems. Sadly, one accident resulted in the death of test pilot Madison and a Ling-Temco-
Vought (LTV) test crew in May 1967, after a loss of tail rotor pitch control from fatigue failure of a critical part during a hover at low altitude.[1397]
NASA Langley took ownership of the fourth XC-142A in October 1968, subsequently flying it until May 1970. The lead pilot was Bob Champine. When these tests concluded, the program came to an end. The Air Force Scientific Advisory Board’s Aerospace Vehicle Panel concluded that, "The original premise that the propeller-tilt wing was well within the state of the art and that it was possible to go directly to operational prototypes was essentially a correct one,” and that the tilt wing "has remarkable STOL capabilities that should be exploited to the maximum.” Indeed, "One of the major advantages of the propeller – tilt wing is the fact that it is a magnificent STOL,” but the panel also acknowledged that, on the XC-142A program, "The technical surprises were few, but important.”[1398]
The results of combined contractor, military, and NASA testing indicated that, as Seth Anderson noted subsequently, despite the XC-142A’s clear promise:
Some mechanical control characteristics were unsatisfactory:
(1) directional friction and breakout forces varied with wing tilt angle,
(2) non-linear control gearing,
(3) possibility of control surface hard-over, and
(4) collective control had to be disengaged manually from the throttles in transition.
Hover handling qualities were good with SAS on, with no adverse flow upsets, resulting in precise spot positioning. Propeller thrust in hover was 12% less than predicted. No adverse lateral-directional characteristics were noted in sideward flight up to 25 knots. In slow forward flight, a long-period (20 sec) oscillation was apparent which could lead to an uncontrollable pitch-up. On one occasion full forward stick did not arrest the pitch-up, whereupon the pilot reduced engine power, the nose fell through, and the aircraft was extensively damaged in a hard landing because the pilot did not add sufficient power to arrest the high sink rate for fear of starting another pitch-up.
STOL performance was not as good as predicted and controllability compromised IGE by several factors:
(1) severe recirculation of the slipstream for wing tilt angles in the range 40° to 80° (speed range 30 to 60 knots) producing large amplitude lateral-directional upsets;
(2) weak positive, neutral, and negative static longitudinal stability; and
(3) low directional control power.
Transition corridor was satisfactory with ample acceleration and deceleration capabilities. Conventional flight performance was less than predicted (11% less) due to large boat-tail drag-cruise.
Stability and control was deficient in several areas:
(1) low to neutral pitch stability,
(2) nonlinear stick force per "g” gradient, and
(3) tendency for a pitch Pilot Induced Oscillation (PIO) during recovery from rolling maneuvers.[1399]
A failure of the drive shaft to the tail pitch propeller in low-speed flight caused a fatal crash that essentially curtailed further development of this concept. The experience of Canadair with the CL-84 Dynavert, a twin-engine tilt wing powered by two Lycoming T53 turboshafts, was in many respects similar to that of the XC-142A. In October 1966, NASA Langley pilots Jack Reeder and Bob Champine had evaluated the CL-84 at the manufacturer’s plant, finding that, "The flying qualities were considered generally good except for a slow arrest of rate of descent at constant power and airspeed that could be of particular significance during instrument flight.”[1400] For a while after the conclusion of the XC-142A
program, the U. S. Navy sponsored further tilt rotor research with the Canadair CL-84, in trials at sea and at the Naval Air Test Center, Patuxent River, MD, looking at combat search and rescue and fleet logistical support missions. Undoubtedly, it was a creative design of great promise and clear potential, marred by a series of mishaps, though fortunately without loss of life. But after 1974, when the CL-84 joined the XC-142 in retirement, whatever merits the tilt wing might have possessed for piloted aircraft were set aside in favor of other technical approaches.