Until electronic navigation systems were developed, pilots also navigated during long flights by using the positions of the Sun, Moon, and stars as explorers on
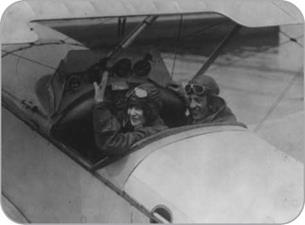
О Early airplanes had open cockpits and flew relatively low to the ground. These factors allowed pilots to navigate by visual landmarks in the days before electronic navigation systems.
|
land and sea had done for centuries. Navigation of this kind is called celestial navigation. Using a device called a sextant, the positions of the Sun, Moon, or certain known stars can be measured to pinpoint an aircraft’s location. Each sighting enables the navigator of an aircraft to draw a line on a map. After several sightings, the point at which the lines cross show the airplane’s position.
Celestial navigation involves making repeated sightings, doing many calculations, and plotting maps. For this reason, the crews of long-range airliners and bombers using this system included a specially trained navigator.
Electronic Systems
A variety of electronic navigation aids have since been developed to help pilots navigate more accurately. The most basic are beacons, or radio transmitters,
The magnetic compass was developed in about the twelfth century in both China and Europe. People noticed that a type of rock called lodestone, when placed on a piece of wood floating in water, caused the wood to turn. It always turned so that one end pointed north. When a magnetic needle is used instead, the needle turns in the same way to line up with the Earth’s magnetic field.
In a simple magnetic compass, the needle is on a dial marked with points of the compass-north, south, east, and west. Because the needle always turns to point north, a person holding a compass can figure out which way to head if, for instance, he or she wants to go west.
A normal magnetic compass does not work well in an aircraft. It swings wildly when the aircraft turns, and it is inaccurate near Earth’s poles. A different type of compass, called a gyrocompass, does not use magnetism. Instead, it uses a spinning wheel called a gyroscope that keeps pointing in the same direction. A gyrocompass installed in an aircraft keeps pointing north, whatever way the plane moves or turns, so it can be used as a reliable compass.
_____________________________________________ /
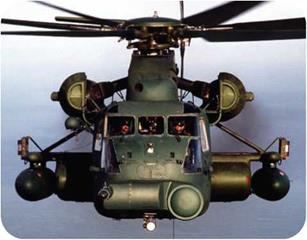
о The MH-53J Pave Low III heavy-lift helicopter is the largest and most powerful used by the U. S. Air Force. It also has very advanced navigational abilities, with an inertial navigation system, terrainfollowing radar, forward-looking infrared sensors, GPS capability, and a projected map display.
|
on the ground. Their positions are marked on navigation maps. A radio in an aircraft picks up radio signals from the nearest beacons and figures out their bearings (the direction of the beacons in relation to the plane). Knowing this helps a pilot to determine an airplane’s position and to steer an accurate course. A variety of systems use radio beacons, including NDB (non-directional beacons), VOR (VHF omnidirectional radio range) and LORAN (long-range navigation). There also is a more accurate version of VOR called TACAN (tactical air navigation) for military aircraft.
When computers became small enough and reliable enough to be carried by aircraft, much more advanced navigation systems became possible. One of these is the inertial navigation
system (INS) or inertial guidance system (IGS). The location of an aircraft is programmed into the system at the beginning of a flight. As the plane flies along, the system detects its movements by using devices called accelerometers. Knowing how much the aircraft has moved, and in which direction, enables its computer to determine the aircraft’s position and to keep track of its progress.
An inertial navigation system can navigate a plane automatically. The system is programmed with the locations during a flight where the plane has to turn. These places are called waypoints. During the flight, the inertial navigation system controls the plane’s autopilot and flies the plane along the planned route from one waypoint to the next.
A modern airliner is equipped with several navigation systems so that if one should fail, another can take over. If everything fails, an air traffic controller
on the ground can guide the pilot or pilots of an aircraft over the radio.